Paper - Transformation of the aortic-arch system during the development of the human embryo (1922)
Embryology - 27 Apr 2024 ![]() ![]() ![]() |
---|
Google Translate - select your language from the list shown below (this will open a new external page) |
العربية | català | 中文 | 中國傳統的 | français | Deutsche | עִברִית | हिंदी | bahasa Indonesia | italiano | 日本語 | 한국어 | မြန်မာ | Pilipino | Polskie | português | ਪੰਜਾਬੀ ਦੇ | Română | русский | Español | Swahili | Svensk | ไทย | Türkçe | اردو | ייִדיש | Tiếng Việt These external translations are automated and may not be accurate. (More? About Translations) |
Congdon ED. Transformation of the aortic-arch system during the development of the human embryo. (1922) Contrib. Embryol., Carnegie Inst. Wash. Publ 277, 14:47-110.
Historic Disclaimer - information about historic embryology pages |
---|
Pages where the terms "Historic" (textbooks, papers, people, recommendations) appear on this site, and sections within pages where this disclaimer appears, indicate that the content and scientific understanding are specific to the time of publication. This means that while some scientific descriptions are still accurate, the terminology and interpretation of the developmental mechanisms reflect the understanding at the time of original publication and those of the preceding periods, these terms, interpretations and recommendations may not reflect our current scientific understanding. (More? Embryology History | Historic Embryology Papers) |
Transformation of the Aortic-Arch System during the Development of the Human Embryo
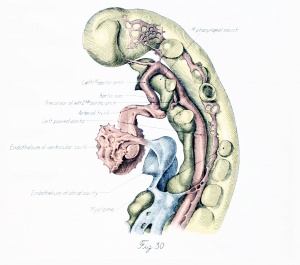
By E. D. Congdon
Division of Anatomy, Loland Stanford Junior University,
and the Department of Embryology, Carnegie Institution of Washington.
(3 plates, 28 text-figures) 47-110.
Introduction
It has been the experience of embryologists that the more carefully the anatomy of the mammalian embryo is studied the more apparent it becomes that the various structures of the body do not in any complete sense recapitulate their phylogenetic history. The form which the recapitulation assumes is by no means precise, since it is much foreshortened and distorted. Because it is so strikingly suggestive of the organization of a gill-bearing ancestor, the system of aortic arches has constituted a favorite illustration for the recapitulation theory; and although it has become evident, through the work of Tandler and others, that these vessels fall far short of repeating their ancestral history, nevertheless all descriptions of their development have been dominated by this theory, and the reader carries away in his memory schemata taken bodily from the branchial-arch system of the anamniotes.
A natural accompaniment to a belief in strict recapitulation was the conception of Rathke (1843) as to the nature of arterial developmental changes. He represented the transformations in the aortic-arch system as being the result of the dropping out of certain definitely fixed segments, as though the system were made up of hard and fast units existing of and for themselves. His well-known diagram has perhaps done more harm than good by forcing implications as to the manner of arterial development that are incongruous with what one actually finds in the mammalian embryo. He left out of account the formative influence of one developing organ upon another, which we are gradually coming to recognize as a factor of great importance. It is being repeatedly demonstrated that the vascular system is especially responsive to the conditions of its environment. A more striking illustration of the influence of adjacent structures could scarcely be found than occurs in the aortic-arch system. During the time that the pharynx, with its pouches, is interposed between the heart and the dorsal aorta, the channels of the arterial blood-stream, in form and position, reflect its relief; but as the pharynx changes its form and the heart descends into the thorax, a new environment is created, which brings about a complete alteration in the branchial pattern and the development of an entirely new arterial arrangement. No precise method of nomenclature for the developing arteries has as yet been evolved. There is lack of precision in using the name given to the adult vessel for the series of short stages of increasing completeness which precede the definitive vessel. The term primitive may be used to call attention to the incompleteness, but frequently, as in the case of the right subclavian, several successive terms would be warranted.
In this study the successive changes in the arch system and the arteries that evolve from it have been followed through human embryos ranging in length from 1.3 to 24 mm. The gaps in the developmental process are small, since 29 stages are included in the series. Microscopic study was supplemented in each case by models made by the wax-plate method. Several of these reconstructions were already in the laboratory, having been prepared in connection with other studies, notably those of Ingalls, Bartelmez, Davis, Evans, and Streeter. Plaster casts were made from some of the plates by Mr. O. O. Heard, whose skilful aid is greatly appreciated. The colored figures were the work of Mr. J. F. Didusch and were drawn from models. I am much indebted to him for their excellent rendering and for further assistance in reconstructing some parts.
I should like also to express my thanks to Dr. C. H. Heuser for his courtesy in permitting the control of the observations on models by a comparison of his beautiful india-ink injections of pig embryos. It is a pleasure to express my obligation to Dr. G. L. Streeter for the interest and encouragement he has shown in this work and for his courtesy in placing freely at my disposal the material and the facilities of the Carnegie Embryological Laboratory.
Branchial Phase of Aortic Arches
In following the growth changes of any structure, it is desirable to have some scale of general body development to which its successive stages may be referred. The myotomes serve the purpose for only a short time. Body-length, though available during the entire period, is unsatisfactory as a criterion, since it shows fluctuations depending upon the degree of development, individual variation, the state of preservation, and the curvature of the body. In table 1 the embryos are arranged in the order of their arterial development, and the age at the end of various developmental phases has been approximated according to Mall's (1912) curve of body-length and age. Because of the large number of embryos upon which the estimates are based, they probably closely approach the correct figures.
The transformations of the aortic-arch system progress through two strongly contrasting phases. The first we may term the branchial phase, since the vessels at this time approximate a pattern which in lower vertebrates is frequently the precursor of the arteries supplying the gill apparatus. The second or post-branchial phase is characterized by the replacement of the branchial by the adult arterial arrangement. For convenience, the breaking of the right pulmonary arch will be
considered as marking the boundary between the two. Though some componentsof the system undergo involution while the arch is still functioning, it is the interruption of the arch that initiates a general disintegration.
Beginning with the establishment of the first arch, the branchial phase lasts about 22 days. The post-branchial period, in the strict sense, endures for nearly 28 years, if this be taken as the growth interval for man. Yet a human embryo of 24 mm. has large arteries in the cranial portion of the body which differ only in minor features from the adult condition, since the vital changes of the second phase are over within two weeks from its beginning.
Table 1. Showing correlation of size of embryos and development of the aortic-arch system
Table 1. Showing correlation of size of embryos and development of the aortic-arch system. | |||
---|---|---|---|
Embryo No. | Length in mm. | Arches present. | Characteristic features. |
Time of establishment of first arch; estimated average length 1.3 mm.; 23d day of development | |||
1878 | 1.3 | I | Slightly plexiform. Presomite stage |
1201 | 2 | I | |
391 | 2 | I | 7 somites |
470 | 4 | I | Neuropores open; 14 to 16 somites |
2053 | 3 | I; II beginning | Anterior neuropore closed; 20 somites; transverse anastomoses between primitive aortae |
Template:CE1201b | 3 | I. II | Earlier mandibular aitery; paired longitudinal neural arteries; |
836 | 4 | II, III | no ventral tract on cord |
Just before establishment of fourth arch; estimated average length 4 mm.; 31st day of development | |||
826 | 5 | III, IV | Earlier mandibular and hyoid arteries |
1075 | 6 | III, IV | Subclavian |
588 | 4 | III, IV | Earlier mandibular and hyoid arteries |
873 | 6 | III, IV | Ventral arterial tract on cord |
988 | 6 | III, IV | |
1380 | 4 | III, IV, pulmonary arches almost complete | |
2841 | 4 | III, IV; one so-called fifth arch; pulmonary almost complete | Early formation of basilar artery |
Just before completion of pulmonary arch; estimated average length 6 mm.; 36th day of development | |||
810 | 5 | III, IV, and pulmonary arches | Late stage in formation of basilar artery, Splitting of aortic sac distinct. Unpaired aorta complete |
1354 | 6 | III, IV, and pulmonaiy arches | |
617 | 7 | III, IV, two so-called fifth arches, and pulmonary arches | Subclavian artery surrounded by brachial plexus. Splitting of sac well marked. Islands at end of basilar artery |
792 | 8 | III, IV, and pulmonary arches | Pulmonary and IV arches widely separated below |
1121 | 11 | III, IV, and pulmonary arches | Right pulmonary artery small; basilar rounded; IV and pulmonary still farther apart |
721 | 9 | III, IV, and pulmonary arches | Cervical segmental arteries becoming interrupted |
163 | 9 | III, IV, and pulmonary arches | Anastomoses of cervical segmental arteries to form the vertebral artery are nearly complete |
Time of interruption of pulmonary arch and of branchial period; estimated average length 12 mm 45th day of development | |||
1771 | 13 | III, IV, left pulmonary and remnant of right pulmonary arch | |
544 | 10 | Vertebral artery complete; identity of arches disappearing; beginning of period of rapid descent of heart and arteries | |
940 | 14 | Definitive aortic arch just taking form. Right dorsal aorta between III and IV interrupted. Remnants still distinguishable. Main pulmonary channel from heart to aorta nearly straight | |
1909 | 15 | Common carotid elongated | |
492 | 16 | Right dorsal aorta distal to IV patent but slender | |
Template:CE74 | 16 | End of period of descent. Definitive aortic arch has curve of large radius. Short segment of right dorsal aorta distal to subclavian drawn out in slender thread | |
End of period of rapid descent of heart and arteries; estimated average length 18 mm.; 50th day of development | |||
1390 | 18 | Definitive aortic arch sharply bent | |
460 | 20 | Summit of definitive aortic arch at superior thoracic aperture | |
2937 | 24 | Sternal bands in contact through most of their length | |
886 | 43 | Origin of right and left pulmonaiy" branches in contact through most of their length | |
Age estimates based on Keibel F. and Mall FP. Manual of Human Embryology II. (1912) J. B. Lippincott Company, Philadelphia. curve of length and age. Table as image Table Reference: Congdon ED. Transformation of the aortic-arch system during the development of the human embryo. (1922) Contrib. Embryol., Carnegie Inst. Wash. Publ 277, 14:47-110. |
Congdon (1922) Table 1 | |||||||||||||||||||||||||||||||||||||||||||||||||||||||||||||||||||||||||||||||||||||||||||||||||||||||||||||||||||||||||||||||||||||||||||||||||||||||||||
---|---|---|---|---|---|---|---|---|---|---|---|---|---|---|---|---|---|---|---|---|---|---|---|---|---|---|---|---|---|---|---|---|---|---|---|---|---|---|---|---|---|---|---|---|---|---|---|---|---|---|---|---|---|---|---|---|---|---|---|---|---|---|---|---|---|---|---|---|---|---|---|---|---|---|---|---|---|---|---|---|---|---|---|---|---|---|---|---|---|---|---|---|---|---|---|---|---|---|---|---|---|---|---|---|---|---|---|---|---|---|---|---|---|---|---|---|---|---|---|---|---|---|---|---|---|---|---|---|---|---|---|---|---|---|---|---|---|---|---|---|---|---|---|---|---|---|---|---|---|---|---|---|---|---|---|
|
Plexiform Origin of Arches
The opponents of the theory of a plexiform origin of the blood-vessels have pointed to the aortic arches as an unassailable example of the correctness of their view. Lewis and others have, however, placed beyond doubt the preexistence of vascular net. The plexus from which the aortic arches develop may cover a widefield or may be jestricted, depending upon the amount of mesenchymal territory available. In the case of the second, third, and fourth aortic arches, this is limited by the small cross-section of their visceral arches. The plexuses preceding the first and pulmonary arches are not so restricted and also have other distinctive features.
The first arch was shown by Lewis (1904) to arise in rabbits from an angioblastic net in company with its ventral connections and the primitive aortae. It was confirmed by Bremer (1912). Evans (1909a) has demonstrated by injection the capillary net preceding it in the duck. In the youngest human embryo of our series (1.3 mm long) the first arch, in its irregular course and in the presence of islands, still gives evidence of its origin from a net. The manner of development of the second, thud, and fourth arches is well illustrated in our material, though the series is not complete for any but the second. One of the first indications of the development of an arch is a slight expansion of the dorsal aorta down into the visceral arch. A similar but more marked projection is seen at the same time pointing caudally and laterally from the common ventral chamber from which the arches arise. This will be termed, for reasons which will be explained later, the aortic sac.
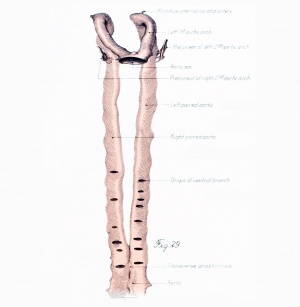
An early stage in the formation of the second arch has recently been studied by Dr. C. L. Davis in a 20-somite embryo. Angioblastic cords and capillaries extend down from the dorsal aorta on one side (plate 1, figs. 29 and 30, drawn from Dr. Davis's models), while on the other an open channel leads ventrally through the arch for a short distance and then goes over into the primitive net. There is also a vessel (not shown in the figures) which extends up from the aortic sac into the visceral arch and ends in the net. Models of three embiyos, of stages ranging from 4 to 17 somites, show beautifully the process somewhat farther along. In two of these a projection from the aorta extends down nearly to the sac, where it ends in capillaries and angioblastic cords. In the other the chief projection is from the sac. It extends upward nearly to the aorta and is separated by a plexus from a short downward-directed sprout arising from the aorta. The appearance of a large channel so soon after the outgrowth of a sparse net is not readily explained as entirely the result of a working over and proliferation of the endothelium of the net. It seems more probable that the development in part takes the form of an outgrowth of the bulging, so that the artery sends out a sprout to supplement the growth activity of the net.
- Through the kindness of Dr. Davis I have had an opportunity to read his finished manuscript and to examine his models and drawings.
A 4-mm. embryo (No. 836) shows the third arch just completed. It is still irregular in caliber and tortuous. As it enlarges, however, as seen in other embryos, the vessel soon becomes straightened and assumes a median position in the visceral arch.
Developing pulmonary arches are in our series frequently represented by independent dorsal and ventral ends (plate 1, figs. 31 and 32). The extension downward from the dorsal aorta lies close behind the caudal pharyngeal complex. 1 Below, a plexus, which earlier can be seen developing caudally from the aortic sac, has given rise to a vessel which has elongated and now extends backward beyond the level of the dorsal sprout, to break up in the pulmonary plexus upon the side of the trachea. The pulmonary arch is completed by an extension of the dorsal sprout which joins the ventral vessel midway in its course, thus dividing it into a proximal portion (now the ventral end of the arch) and a distal portion (the primitive pulmonary artery). Further observations bearing on the development of this arch and the earlier studies on this subject will be referred to in the description of the development of the pulmonary artery.
The pulmonary arch is more variable as regards the position of its distal end than are the others. As it enters the aorta it may be separated by a distinct interval from the fourth arch (figs. 6, 7) or may be close to it; a common upper end of the two also is frequent. These variations are dependent in part upon changes in the caudal pharyngeal complex, which sometimes lies so near the aorta as to prevent the two arches from close approximation, while at other times it is withdrawn more ventrally. The vagus nerve and its recurrent branch also limit the territory open for occupation by the pulmonary arch on its caudal side, since they pass close behind the caudal pharyngeal complex.
There have been several studies on the development of the second and succeeding aortic arches by both the reconstruction and the injection methods. The second, third, and fourth arches were found in the rabbit by Bremer (1912) to be preceded by a vascular plexus from the ventral aorta. He described this as potentially double for the second arch and multiple for the succeeding arches. Sabin (1917) figures irregular double channels for the second arch in injected chicks.
In human embryos simple loops (figs. 2, 3), of greater than capillary caliber, not infrequently come off from the aorta at the upper end of the visceral arch before any definite sprout has become established. They may remain for a time as a part of a completed vessel, where they are usually referred to as "island formations." They were found most frequently in the pulmonary arch, but were also seen in the second, third, and fourth arches. Occasionally they were found in the ventral end of the arch. Lewis (1906), in his discussion of the fifth arch, pointed out that they are of general occurrence in mammals. A survey of the literature on the lower mammals serves to confirm this, and it may be assumed that it is true also of man. It is possible that these loops may be expressions of a tendency toward a double channel in the visceral arches, such as Bremer describes.
- 1 This term is applied by Kingsbury to the entire pharyngeal evagination on either side, which lies caudal to the third pharyngeal pouch.
Successive Development of Arches and Shifting of Current
The existence of aortic arches is the result of the interposition of the pharynx, with its pouches, in the path of the blood-stream from heart to dorsal aorta. Since the arterial end of the heart at first lies below the cranial end of the pharynx and later shifts backward relative to it, the aortic arches develop in regular order from before backward. As the more caudal ones are completed, the first and then the second undergo involution. Later, the third arches cease to carry part of the aortic stream. The current from heart to aorta is in this way shunted caudally. Successive stages in the process are represented in figures 1 to 16.
The earliest channel is the first arch, which for a time carries the entire aortic current. It curves dorsally in a groove behind the head process in the mandibular arch. At first it faces forward, but with the increasing curvature of the head region it becomes more and more exposed to ventral view.
In an embryo of 3 mm. (No. 2053) a second arch is forming (fig. 1, and plate 1, figs. 29, 30.) In the next individual of the series (No. Template:CE1201b) the second arches are well developed and the first have already decreased greatly in caliber (fig. 2). The next available stage has a large third arch and a dwindling second (fig. 3). Models were made from 8 embryos in which the fourth but not the sixth arch has developed. In all but the youngest of these the first arch has gone and only a slender channel passes through the mandibular arch. In the more mature specimens the second arch also has disappeared (figs. 4, 5). The hyoid arch is now occupied by a channel too slender and tortuous to be regarded even as a remnant of an aortic arch. The phase in which the fourth arch is the most caudal feeder to the aorta begins with embryos averaging about 4 mm. in length and ends with embryos averaging 6 mm. The succeeding portion of the branchial period, which is characterized by the presence of a pair of pulmonary arches and is terminated by the interruption of the right arch, is represented by embryos from about 6 to 12 mm. in length. The approximate length in days of the various divisions of the developmental period can be obtained from table 1.
During the branchial period the changing bed of the stream from heart to aorta follows these successive paths: first arch, first and second arches, second and third arches, third and fourth arches, third, fourth, and sixth arches, and, not rarely,
the latter three in company with the so-called fifth arch. It is possible that the first, second, and third arches also for a time share the current, though this condition was not observed in our series. For most of the interval before the completion of the fourth arch, a single pair of vessels carries the greater part of the bloodstream, so rapidly do the first and second arches dwindle. In the later part of the branchial period, covering 9 of approximately 22 days which constitute the total branchial span, there is comparative stability in the arch system, while the current is divided between the third, fourth, and pulmonary arches.
The length of the arches is surprisingly fixed during their entire existence, although the body more than doubles in length during the same interval. The length of the third and of the fourth arch was measured on models of 4 embryos in of the arches to elongate is due to the lack of active growth in their immediate environment (the caudal portion of the pharynx) at this time, and this in turn is an expression of the regressive changes which the organ undergoes.
The chief cause of the disappearance of the first and second arches is probably to be found in the shift of the blood-stream to the more caudal arches, which accompanies the caudal movement of the aortic sac. The rapid growth of the propharynx, 3 in both width and length, doubtless hastens their degeneration by increasing the length of their course.
So-Called Fifth Arch Morphology of Pulmonary Arch
There are two vascular types that appear in descriptions of the so-called fifth aortic arch in mammals, and both occur frequently in man. One is the islandformation of the upper end of either the pulmonary or fourth arch, the other is a channel connecting the fourth and pulmonary arches. Most frequently this vessel comes from the proximal end of the fourth arch, or the subjacent aortic sac, and enters the pulmonary arch above. Its upper end sometimes enters the fourth arch. It may be represented only by spurs corresponding to its extremities. The islands at the upper end of all of the arches but the first and at the lower extremity of some of them have already been referred to and interpreted as retained parts of the plexus which precedes the arches (fig. 4). They require no consideration in a discussion of the fifth arch.
Models of 7 embryos in which the pulmonary arch was almost or just completed were available. Among them were found 3 well-developed vessels arising from the aortic sac or fourth arch and ending above in the distal end of the pulmonary arch (figs. 8, 18, 22). One was of much smaller diameter than the arches, but another was as large as the fourth arch. They all lay in deep grooves of the caudal pharyngeal complex. Arterial sprouts corresponding to the ends of these vessels were found in relation with many of the other caudal pharyngeal complexes and usually can be shown to lie in corresponding though more shallow grooves. The propriety of regarding these channels as rudimentary fifth arches is still a matter of debate after the passage of nearly forty years since Van Bemmelen (1886) claimed their existence in mammals and in spite of the work of nearly a score of investigators. Tandler (1909) was the first to describe them for man, and figured vessels similar to those observed in our series, except that they had a somewhat longer dorsoventral course. He also found spurs corresponding to their ends. He believed that these constitute true fifth aortic arches, but regarded them as very transitory. Only 6 instances of the complete vessels in man have been described up to this time. More than 20 have been found among the lemur, mole, rabbit, cat, guinea-pig, and pig.
It was a corollary to the principle that embryonic blood-vessels depend greatly upon their environment for their form that Lewis (1906), in a study of rabbit and pig embryos, denied the authenticity of so-called fifth aortic arches, on the ground that the existence of fifth visceral arches had never been proved. Kingsbury which the fourth arch had been very recently completed, and also of 4 at the beginning of the post-branchial period, when the arches were about to lose their identity. The measurements were divided by the magnification of the model and corrected approximately for shrinkage. Between the two periods the average length of each showed a negligible increase of less than 5 per cent. The failure
- 3 Kingsbury distinguishes the cranial portion of the pharynx, including the second visceral arch, by this term, and calls
the more caudal part the metapharynx. The propharynx grows more rapidly in length and width than the caudal division.
Figs. 1 to 16. Ventral views of aortic-arch system, showing successive developmental stages. In the earliest stage only the first arch is present, while in the last (a full-term fetus) the vessels have acquired nearly their adult form. The so-called fifth arch is indicated by asterisk. Figure 1, embryo No. 2053, length 3 mm.; figure 2, embryo No. Template:CE1201b, length 3 mm.; figure 3, embryo No. 836, length 4 mm.; figure 4, embryo No. 588, length 4 mm.; figure 5, embryo No. 1075, length 6 mm.; figure 6, embryo No. 1380, length 6 mm.; figure 7, embryo No. 810, length 5 mm.; figure 8, embryo No. 617, length 7 mm.; figure 9, embryo No. 792, length 8 mm.; figure 10, embryo No. 1121, length 11 mm.; figure 12, embryo No. 1771, length 13 mm.; figure 13, embryo No. 940, length 14 mm.; figure 14, embryo No. Template:CE74, length 16 mm.; figure 15, embryo No. 1390, length 18 mm.; figure 16, full-term fetus.
(1915a), in his study of the development of the human pharynx, points out that the nature of the components of the caudal pharyngeal-pouch complex, exclusive of the fourth pouch, is still too uncertain to justify the claim of a fifth visceral arch.
He finds, however, that in the human embryo possible fifth pouches may reach the integument. Whether they are rudimentary fifth arches or not, there seems to be warrant for considering these structures as more homogeneous and definite in character in man than has been generally recognized. The residue left after the islands are eliminated consists, for the most part, of channels passing from near the dorsal end of the pulmonary arch to the proximal end of the fourth arch or the adjacent aortic sac. The chief variation from this type is offered by vessels that terminate distally in the fourth arch. The sprouts lying in grooves of the caudal pharyngeal complex and otherwise having the same relations as the ends of these channels may be regarded as incomplete stages of the same type. Their frequency, taken with that of the complete channels, was found to exceed 50 per cent.
The so-called fifth arch is described by several authors as arising later than the pulmonary. In the human embryo, at least, it will require further data to determine the time relation between the two vessels. The difficulty lies in the lack of a
precise period at which we may regard an arch as coming into existence, owing to the gradual nature of its development from a plexus. Nothing is known of the manner in which the so-called fifth arch disappears. Certainly it does not retain its individuality long, since it has not been described in older mammalian embryos. As one follows the deep-seated changes of the parts of the arch system from which the aortic arch and pulmonary artery are formed, it becomes easy to picture its early interruption and the taking up of more or less of the material of its wall in these larger vessels. It may be that some of the spurs which have been described in this region are stages in the development, while others are steps in the regression, of the so-called fifth arches, and it is very likely that the transition from the former to the latter is frequently accomplished without the establishment of a complete channel.
Fig. 17. Development of the pulmonary artery and ductus arteriosus, showing degeneration of distal part of right arch and the incorporation of its proximal part into right branch of pulmonary artery; also approach of right and left branches through wall of pulmonary stem, a, 7-mm. embryo, No. 617; b, 11-mm. embryo, No. 1121; c, 13-mm. embryo, No. 1771; d, 18-mm. embryo, No. 1390; e, 43-mm. embryo, No. 886.
Shaner (1921) states that in vertebrates it is not rare for the sixth arch to develop, after the fifth is established, as a shorter vessel coming off from both ends of the fifth. The intermediate segment of the fifth then disappears, leaving its extremities as parts of the so-called adult sixth. This suggests a possible significance in the fact that in man the so-called fifth arch enters the pulmonary arch close to its upper end. Of the 6 well-developed so-called fifth arches that have been
described in the human embryo, 5 enter the pulmonary near its termination. If it be established that these vessels are true fifth arches, their usual termination would indicate strongly that the upper end of the pulmonary arch is the homologue of
the distal portion of the fifth.
Not only is the status of the channel lying between the fourth and last aortic arches unsettled, but the pulmonary arch also depends on a more complete understanding of the caudal pharyngeal complex for its interpretation. Shaner has recently shown that in the turtle the terms sixth arch and 'pulmonary arch are not necessarily synonymous. He finds an arch caudal to the fifth, which gives off the primitive pulmonary artery but still is not the equivalent of the human pulmonary arch, since it lies craniolateral instead of caudomedial to the caudal pharyngeal complex. At the same time the equivalent of the human pulmonary arch is indicated by a spur from the upper end of this vessel curving around to the caudomedial side of the complex.
Ventral Connections of Aortic Arches - Aortic Sac
The literature concerning the nature of the ventral connections of the heart and branchial arterial arches shows a surprisingly great diversity of view, considering the numerous accounts of vascular development. The terminology of this region is in a correspondingly unsatisfactory state. Few authors are in complete agreement in the use of such fundamental terms as aortic trunk, bulb, or ventral aorta, and we still find in recent editions of our anatomical texts portions of the paired dorsal aortae referred to as parts of aortic arches, as in the time of Rathke and von Baer.
In the mammalian embryo a saccular enlargement intermediates between the aortic arches and trunk. A slight swelling can be made out at the junction of the first arches and trunk in the human embryo even before the second arch is established (fig. 1). It reaches its highest development when giving origin to the third, fourth, and pulmonary arches and before it has begun to separate into its aortic and pulmonary divisions (figs. 5, 6). At this time it is decidedly flattened dorsoventrally and the arches radiate from it. It varies greatly in form, corresponding to the tendency of this region to be drawn out in either its craniocaudal or transverse axis, and also in response to fluctuations in the form of the individual pouches and arches. The cleft between the points of origin of the fourth and pulmonary arches begins to deepen soon after the caudalmost arch is completed. Before the branchial stage is at an end the sac has separated completely into aortic and pulmonary portions. The pulmonary division is tubular but the part that gives rise to the third and fourth arches is for a time still somewhat flattened and saclike.
The enlargement at the origin of the arches is not confined to mammalian embryos. Greil (1903), in his work on the development of the truncus arteriosus in Anamnia, finds a similar chamber in Acanthias embryos and Salamandra larvae. It is also encountered in certain adult gill-bearing vertebrates. Rose (1890) figures it in his study of the heart in the ganoid Polypterus bichir and the urodele amphibian, Sieboldia maxima (Cryptobranchus japonicus). Dr. Harold Senior tells me that in the American form, Cryptobranchus alleghenesis, the enlargement is present, but the common cavity is much restricted by the medial extension of septa between the openings of tin' arches.
His (1880) and Bujard (1915) have recognized the existence of a ventral aortic swelling in the human embryo and designated it aortic bulb. Gage (1905) and Jordan (1909) termed it the aortic sinus. Griel and Rose did not devote especial attention to the sac in their studies of gill-bearing vertebrates and gave it no name. In the adult fish and amphibian it is doubtless to be classed as an aortic bulb, though these non-muscular enlargements distal to the heart do not usually give off the arches directly. In this paper the specific term aortic, sac (saccus aorticus) will be used for the embryonic enlargement. This is meant to include not only the chambers between the arterial trunk and the arches, but also the reduced sac distal to the aortic trunk, which persists for a time after the pulmonary trunk has become separated off.
On looking "for an explanation for the expansion at this point it is necessary to determine the relative importance of adaptation to function, such as is found throughout the adult circulatory system, and of factors peculiar to the developmental period. The aortic bulb of adult fish and amphibia probably shares with the elastic mammalian aortic arch and other large arteries the function of distributing the systolic pressure over a large portion of the arterial circle.
Stahel (1886) claims that an enlargement of the portion of the human aortic arch opposite the emergence of the innominate, carotid, and subclavian arteries is a response to the added strain on the wall at this point resulting from the sudden
deflection of part of the current into these vessels. Thoma does not accept this explanation. It is possible that the embryonic aortic sac is the result of the combined action of these two principles. Yet it must be remembered that the embryonic chamber differs greatly in its nature from the adult bulb and arch. As to its makeup, we can say with certainty only that it consists of an endothelial sac, though histogenetic study may well show that myoblasts and fibroblasts are already to be reckoned with. In any case its wall is very thin. It follows the relief of the ventral pharyngeal wall ; it is a cast of which the pharyngeal surface is a mold. If we are to consider the embryonic sac as serving as an elastic reservoir similar to the adult bulb and aortic arch, it is necessary to recognize the support afforded by the pressure of surrounding resistant organs, exerted through the intermediate mesenchyme, as, for example, the pharyngeal endoderm above and the atria of the heart below.
Kingsbury (1915a) noted that the arterial channels ventral to the pharynx, acluding the aortic arches, fitted snugly into concavities of the pharyngeal wall, he concluded that the vessels exerted a molding influence upon it. It is c \nlt to say just how much of the channeling of the pharyngeal surface is due p\ e arteries and how much to other factors. Doubt is cast upon a preponderating influence of the blood-vessels by the fact that the grooves on the ventral floor of the pharynx, filled for a time by the first and second aortic arches, do not disappear when these vessels are lost.
An entirely different explanation for the presence of the sac has been suggested by Dr. Streeter. He has observed that it is a characteristic of early vessels, well illustrated by the early dorsal aorta, to have a diameter much greater proportionately than would be required for the adult vessel. He suggests that this may be due to proliferation of reserve endothelium which a little later will be used in the rapid differentiation of the vascular system.
The connections of the aortic arches with the arterial or aortic trunks are termed paired ventral aorta? in most text-books of human anatomy, and the schemata which they contain correspondingly show the arches arising from a pair of longitudinal ventral trunks. As has been stated, a few investigators have recognized the error of this description by using the term bulb or sinus. While the arterial blood in the human embryo passes from trunk to arch by an unpaired sac, there are certain temporary channels to single arches which, by their cranio-caudal course, resemble fragments of a ventral aorta. Such are the longitudinal ventral segments which appear in the later history of the first and second arches and the paired ventral sprouts which for a time run caudally from the pouch before they take on a more transverse direction as part of the pulmonary arches. One might even include the primitive ventral arterial twigs of the subpharyngeal regions, which have the position of ventral aortse in the region of the first and second aortic arches, though at a time when the arches have already disappeared. These various more or less longitudinal elements are rightly to be regarded as indications of a general structural plan common to higher and lower vertebrates, but carried on in some of the latter to a completeness which admits of the existence of paired ventral aortse. However, these considerations certainly offer no justification for the use of the term ventral aorta:, in man, since such vessels are not to be found at any stage of his development.
Involution of First and Second Aortic Arches - Origin of Stapedial and External Carotid Arteries
In the region below the propharynx there is a period of instability and of readjustment of the vascular channels after the disappearance of the first and second aortic arches. Our study of this period is based on but few models, since only vessels turgid with blood or good artificial injections can be relied on to demonstrate the change of the arches into a plexus and the beginning of the arteries therefrom.
Soon after the third arch is established, the first has given place to a tortuous and much more slender channel (fig. 3). It is best developed at the upper end of the visceral arch and is usually lost in the plexus at the lower end. There is often rf
distinguishable close to the vestibule an arterial sprout occupying the position c ira the ventral end of the arch before its disappearance (figs. 3 to 9). After the fourth arch is complete, a similar channel is found to have replaced the second t
this also is usually lost in a plexus in the subpharyngeal region. These ves.' clearly not to be regarded as late stages of the arches. They have not the size or form of the arches. Functionally, also, they differ. Since they are interrupted below, evidently their current is usually downward from the dorsal aorta?. They serve to supply the substance of the visceral arches and not to convey the bloodstream from heart to aorta.
The vascular successors of the arches remain but a short time and are in turn replaced by slender vessels, which, except near their origin from the dorsal aorta?, are scarcely more than capillaries. These run close to the caudal confines of the first and second visceral arches. These two pairs of successive vessels may be termed, respectively, the earlier and later hyoid and earlier and later mandibular arteries from the visceral arches which they supply. The later hyoid and mandibular arteries are both present in the period between the establishment of the fourth and sixth arches. In the branchial period, after the completion of the pulmonary arch, the upper end of the later hyoid vessel seems always to be present. It is still clearly distinguishable in the post-branchial period (plate 3, figs. 37 to 39). It is the equivalent of the stem of Broman's (1898) hyostapedial artery in man. Tandler has described in detail the development of the stapedial artery in the rat by the
capturing of branches from the upper end of the first arch by the upper end of the second arch. There can be little doubt that the "arches" he refers to are the earlier or later mandibular and hyoid arteries of the foregoing account. He finds that the upper end of the "second arch" moves caudally a short distance along the dorsal aorta. This we recognize as the later hyoid artery, which we know has a slightly caudal position as it comes off from the dorsal aorta, due to its passing down the caudal side of the visceral arch. In 13 and 14 mm. human embryos this vessel has increased in caliber, keeping pace with the expansion of this region in connection with the development of the ear. Tandler also finds it in the human and identifies it as the stapedial artery. The development of its branches and its later history were not followed in the present study.
At the time the stapedial artery is developing in the hyoid arch, the precursor of the external carotid is taking form on the ventral side of the propharynx. While the second arch is disappearing, a pair of symmetrical arterial sprouts is usually distinguishable, extending forward from the aortic sac in the region earlier occupied by the ventral segments of the first two arches. In the two specimens showing this stage these sprouts he ventral to the thyroid gland, and in one of these the distal branches of the right sprout have been captured by the opposite vessel. Later (fig. 3) , after the second arch has gone, these ventral primitive arteries are found to be on either side of the thyroid gland. Each sends out a ventral branch to the plexus of the pericardium and integument, and also a dorsal branch, which either breaks up in the rich plexus of the thyroid gland or extends for a variable distance through the subpharyngeal plexus toward or into the base of the mandibular or
hyoid visceral arches (fig. 4).
An interesting feature of the adjustment of the ventral pharyngeal vascular channels is the occurrence of small vascular enlargements in the subpharyngeal plexus or at times in the ventral primitive arteries. These are termed lacunae by Tandler (1902) and Lehman (1905). In the figures of Lehman they are represented as being independent of the circulatory system; she regarded them as fragments left behind by the involution of the first and second arches. Dr. Streeter suggests that they may be proliferations of endothelium for the supply of the developing ventral arteries, and thus progressive rather than regressive in nature. No evidence for the degeneration of the endothelium of the two arches was found. It seems probable, therefore, that it is worked over into the capillary net and the larger vessels that succeed them. The regression of small vessels will be considered again with reference to the interruption of the segmental arteries during the formation of the vertebral.
In the post-branchial period the differentiation of the subpharyngeal region has permitted the ventral artery to develop branches somewhat resembling those of the definitive external carotid. There are, for example, lingual twigs passing between strata of the developing lingual muscles. The artery is now sufficiently withdrawn from the thyroid plexus to have a definite thyroid branch. Other ramifications are already present, and there also may be finer branches given off from the third arch close to it. While the vessel is thus taking form, it is gradually withdrawn from the midline. At the end of the branchial developmental phase it is given off from the third arch near its junction with the aortic sac (fig. 12).
The process of involution of the two cranial aortic arches and of the development of the arteries that succeed them has been variously interpreted. The earlier observers did not find the mandibular and hyoid arteries. As material improved and experience increased, these vessels were usually seen only in part and were interpreted as fragments, due to the breaking down of the corresponding arches, rather than as vessels that had taken their place. The point of first interruption has been placed at either end or at some intermediate point, depending probably on the chance conditions of distention of parts of the arteries rather than upon individual or specific differences among mammals. A further study of these changes of vascularization by the injection method is highly desirable.
Post-Branchial Phase
(Including embryos up to 25 mm in length.)
The disappearance of the aortic-arch system is amply explained by the separation of the outflow from the heart into two streams and by the changes in the environment of these due to the shifting of the organs among which they must find their way. Though it is necessary, for convenience, to describe the arterial evolution by stages, and to a certain extent independently of the movements, it must not be forgotten that it is a gradual process and is paralleled step by step by changes in the surroundings.
During the disintegration of the branchial arterial pattern, some of the arches and their connections may be identified for a time; but since their distinguishing characters are largely topographical and their walls differ but little in structure, their individuality is gradually lost and their material worked over and increased to form the arteries which succeed them. The difficulties encountered in tracing their later history are paralleled in the study of the development of other tubular systems, as, for example, the hepatic and pancreatic ducts, or the Wolffian ducts in connection with the urogenital sinus. To follow the material derived from them to its position in the post-branchial vessels, it is necessary to know whether there is, during growth, a fusion or a splitting at the point of bifurcation of vessels, and whether changes in the interval between two lateral branches are due to an alteration in the length of an intervening portion of the main stem or to a more complex shifting of the material by which the branches move bodily along the wall.
The task of tracing the material of the arch system into the vessels of the postbranchial period is well worth while, not because we expect them to take part as distinguishable units in the adult vessels, but because, on account of the definiteness and multiplicity of the arches and their connections, they are especially good material for gaining some conception of how rapidly vascular territories in general lose their identity and to what degree their material is intermingled with adjacent regions during development. The history will, at best, be incomplete, since the largest embryo of our series, though its form is far along toward the adult condition, is but 24 mm. in length and must increase about seventy fold before the adult dimensions have been reached.
The breaking up of the arch system of the late branchial period, with its 3 pairs of arches, is made possible by its interruption in four regions. This is preceded by a movement of the arches as far caudally as their pharyngeal pouches and other structures allow. The time occupied for each interruption is brief; it can be roughly estimated as a day. The left pulmonary arch is the first to disappear, thus permitting the evolution of the pulmonary vessels. The dorsal aorta on each side, between the third and fourth arches, next loses its continuity. This is of especial help in the formation of the definite aortic arch and the innominate and common carotid arteries. Finally, the dorsal aorta, by its interruption close to its caudal end, prepares the way for the remolding of a large part of the right paired aorta, together with the right fourth arch, into the subclavian artery of this side.
The involution of the right pulmonary arch is confined to the part distal to the origin of the right primitive pulmonary artery (fig. 17, a to d). Models were made of the arch system of 2 embryos in which this region was in a condition of reduced diameter preliminary to its interruption, at the time when evidences of the causes of its degeneration should be most apparent. In fact, indications are not lacking of the presence of mechanical conditions that might cause its involution. The arch seems to be pulled caudally at its ends and held back in its middle portion by the vagus nerve and its recurrent branch. Both ends are bent somewhat caudally and are smaller in diameter than the intermediate part. The upper end comes off the aorta at about the same angle as found at this time in the more craiial segmental arteries, where it is clearly due to the caudal shifting of the aorta relative to the surroundings.
The existence of a caudal and a transverse pull upon the proximal end is indicated not only by a caudal slope of this segment just where it passes down to the origin of the primitive pulmonary artery but also by the rapid withdrawal caudally and to the left of this origin after the segment is broken. The intermediate part of the interrupted segmeit lies closely applied to the cranial surface of the loop formed by the vagus and its recurrent branch. The arch, in the character of its curve, shows molding by the nerve, and frequently the aorta just caudal to it is flattened. The molding is still more clearly seen on the left pulmonary arch, which does not become interrupted.
In spite of indications of pressure from the vagus on the degenerating arch, models of two embryos in which the arch is not reduced do not show any considerable flattening of the vessel walls against one another due to pressure. The lumen is
rounded, and in one specimen, in which the mesenchymal layer of the wall can be made out satisfactorily, this is much thickened. The first distinguishable step in the reduction, then, is a contraction.
The disappearing segment of the arch seems to have been exposed to unfavorable conditions in regard to both longitudinal tension and pressure by the vagus nerve. Yet a comparison of the history of the right and left arches at this time brings out clearly that these factors are not the exclusive cause. The left arch shows a well-marked molding by the vagus and its recurrent branch, but it does not retrogress; on the contrary, at this time it is increasing in diameter. The reason for its persistence in spite of unfavorable surroundings is probably to be found in its more advantageous position relative to the pulmonary current. The bifurcation between the pulmonary trunk and the arches is weil to the left of the mid-sagittal plane, due to the presence of the aortic trunk on the right. In consequence, the left arch has a much shorter and more direct route to the dorsal aorta than the right, thus receiving more blood and being better able to maintain itself.
One embryo, in which the arch as a functioning element had gone, still had a cellular cord extending from the junction of the right pulmonary artery and the persisting ventral segment of the arch to the ventral edge of the caudal pharyngeal complex. Though its cross-section was made up of a number of cells, the endothelial and mesenchymal elements could not be distinguished from each other. The post-mortem changes in the surrounding tissue made it impossible to determine whether or not its cells were degenerating before the death of the embryo.
We are fortunate in having models of three stages in the breaking of the dorsal aorta between the third and fourth arches. In the first, a continuous curvature of the third arch and the aorta cranial to it had developed, while the fourth arch had similarly formed a common arch with the aorta on its caudal side (figs. 9, 11 ; plate 2, figs. 34, 36). This indicates that, as the current in the fourth arch passes caudally, that of the third arch moves in a cranial direction. With the perfection of these curves, the intermediate aortic segment becomes more slender (fig. 12) and its ends are pulled slightly downward and away from each other to give it an arched form. It shows contraction by a thickening of its wall and decrease of its lumen.
Lehmann describes a condition in the pig (missing in our series) in which the further moving apart of the distal portions of the two arches results in the pulling out of the intermediate segment to a mere thread. In our next stage this filament is probably broken, as we find a rounded mass at the upper end of the fourth arch, evidently due to the retraction of its mesenchymal sheath (plate 3, figs. 37 to 39) . The endothelial core was traced backward through a few sections as a solid rod. The anterior end of the degenerating vessel was not found.
If tension in connection with the caudal shifting of the aorta plays a causal role in the disruption of the pair of aortic segments, it seems to be secondary in importance to a decrease in the current-flow. The contrasting curves of the third and fourth arches, before the segment has stretched perceptibly, indicate that the current is passing from them to the aorta in opposite directions, and consequently the stream in the disappearing segment is nearly at a standstill.
The interruption of the caudal part of the right paired aorta takes place in a manner very different from that indicated by current figures and descriptions. These err in representing the obliteration of a long segment of the vessel. There is, in fact, great economy of material in this operation, since only an insignificant terminal segment actually disappears. Before it has been especially affected, the entire right paired aorta, as far forward as the fourth arch, becomes reduced in diameter, so that it retains a lumen adequate only for the supply of the subclavian. Decrease in current here seems to be the primary cause of involution, as in the case of the pulmonary arch. Here, also, the left counterpart persists, having a larger current. The cause of the falling off of the current of the right vessel relative to the left is probably to be found in changes that have come about in the pulmonary aortic trunks at this time. As has already been explained, the pulmonary trunk is now throwing its current entirely into the left paired aorta. The aortic trunk also, in the two embryos that were studied, has taken an oblique direction, well marked later, and is therefore sending more blood into the left than into the right fourth arch. The greater part of the right paired aorta caudal to the fourth arch retains a diameter equal to the subclavian. The short caudal end distal to the subclavian shows further contraction by a narrowing of its lumen and a thickening of its wall. Later, as the aorta shifts caudally, it is stretched out into a filament over 3 vertebral segments in length (fig. 14). This is made possible by the fixation of the more caudal part of the paired aorta by the right subclavian and its branch, the vertebral, which thus fastens it to the vertebral column and to the surrounding tissues.
The different interruptions here described seem to have much in common and are due to the same factors that brought about the involution of the first and second arches. In each instance there is a preliminary decrease of current-flow, though its cause in the unpaired and symmetrical segments is dissimilar. It seems probable that longitudinal tension, resulting from the caudal shifting of the heart and aorta, serves to augment the effect of the change in current. At an early stage there is lacking clear proof of tension, such as would be furnished in the case of a stretched rubber tube by the narrowing of its wall and lumen. The first decrease in caliber was due to a contraction of the vessels and was therefore accompanied by a thickening of the wall. The response of the artery to the tension and other unfavorable influences was vital in its nature and not merely physical. It was only after their walls weakened that the aortic segments were rapidly pulled out into filaments. The pressure of the vagus nerve probably assisted in the involution of the left pulmonary arch. Here, again, direct proof of its action, which in this case would be a marked lateral compression of the degenerating vessel, was lacking. There was no available material in which to study the degenerating first and second arches for evidences of unfavorable effects of tension.
Before considering in detail the manner in which the large vessels derived from the arch system take form, it might be well to become familiar with a stage midway between the late branchial and the approximately adult condition found in a 24-mm. embryo. In plate 3, figure 38, showing a 14-mm. embryo, it can be seen that the right half of the aortic sac is represented approximately by a transverse tube, concave cranially, and making, with the modified left limb of the sac and the derivatives of its third arches on either side, the arm of a candelabrum-like figure the upright stem of which is the aortic trunk. From the tube on the right and the sac on the left arises a vessel, which still bears some resemblance to the third arch, and also a derivative of the fourth arch. These vessels, however, take origin more laterally and dorsally, relative to their surroundings, and run more directly dorsal than do the arches in the branchial period. The upper end of the zone arising from the fourth arch is still marked on both the right and the left side by the tapering remnant of the interrupted dorsal aorta as ear her described. The tube of the right side and its fourth arch derivative are much longer than their equivalents on the left side, whereas the latter are of much greater diameter. Those on the left also he almost a vertebra length more caudally.
The definitive aortic arch is already roughly outlined at this stage, and the left half of the sac and the widened left fourth arch are parts of it. The tubular derivative of the right half of the sac may be termed the primitive innominate artery, and the regions corresponding to the lower parts of the third arches, up to the origin of the primitive external carotid arteries, are the primitive common carotids. Distal to this point are the primitive internal carotids.
Individual variation must be reckoned with always in describing a single embryo as a type. In this instance the model of an embryo slightly older than our 14-mm. specimen, while also normal in appearance, shows a marked difference in the proportions of the innominate and right common carotid. The innominate has still the form of a slightly elongated half of the aortic sac. To compensate for this the common carotid is longer than in the other embryo.
The pulmonary vessels no longer show any element suggesting the proximal segment of the right pulmonary arch. The main pulmonary channel is a single large straight vessel leading to the distal end of the definitive aortic arch and giving off a pair of pulmonary arteries near its origin. The right paired aorta, though not interrupted at its distal end, is much smaller than its counterpart on the left side. The subclavian arteries are given off from the paired aortae just before their confluence to form the unpaired aorta. The vertebral arteries are present as branches of the subclavians, and the basilar is completed through most of its later course by the fusion of the longitudinal neural arteries. In position the arch system is now about midway between its earliest location in the occipital region and its ultimate position in the thorax.
The central feature in the post-branchial arterial development is the evolution of the aortic arch. It comes into being from various sources. Its beginning is indicated by the replacement of the left fourth arch and the dorsal aorta between it and the left pulmonary by a tube of continuous curvature at the time the aorta cranial to it is narrowing its lumen preparatory to obliteration. In the arterial system of a 14-mm. embryo such as has just been described (plate 3, figs. 37 to 39), angles and inequalities of diameter block out roughly the arterial regions which are losing their individuality in the formation of the arch. These are the aortic trunk, the tube derived from the left half of the aortic sac, the left fourth arch, the left dorsal aorta between the fourth and pulmonary arches, and, finally, that portion of the left paired aorta lying distal to the pulmonary arch. The irregularities of the arch have disappeared by the time the embryo reaches a length of 17 mm.
The radius of curvature of the early aortic arch changes in connection with alterations in the direction of the long axis of the heart as it shifts downward into the thorax. While the arch is in the lower neck region and the ribs of the two sides have
not become united in front by the rudiments of the presternum and sternal bands,
the curve of the arch is rather open, though it will be seen that its radius is already
less than when first forming (figs. 20, 24). As the heart passes into the dorsal concavity of the thorax and is encircled by the ribs, its apex points less ventrally
and more caudally. In consequence the pars ascendens of the arch assumes a more
longitudinal direction. Since the more distal part of the arch is held by a number of
branches, a sharp bend develops between the two at the origin of the innominate and
left common carotid. By the time the summit of the. arch has reached the level of
the first thoracic vertebra and the rudiments of the sternum have fused to complete
the superior thoracic aperture, the pars ascendens is nearly aligned with the long axis
of the body, and the arch for the time has more the form of a letter V than of a segment of a circle (figs. 21, 25).
The arch is also peculiar at this time in that it lies almost completely in the midsagittal plane. This is because the dorsal aorta has not yet moved to its position at the side of the vertebral bodies, which are at this time so immature as not to have assumed the strong convexity which later characterizes them in this region, and the heart has not yet taken on its obliquity relative to the long axis of the body.
The tracing of the regions of the arch system into the later arteries, as well as an understanding of the changes in the latter, is largely a mattei of inference based upon changes in dimensions. Accordingly, the length and circumference of various parts of the arch system were obtained, as also the length and circumference of the parts of later vessels with which they were to be compared. For the study of most regions a series of 11 embryos of graded development were used. Of these, 6 represented the branchial stage and 5 the post-branchial. The measurements were made on models and then reduced to their true value by dividing by the magnification. The reliability of the data was considerably increased by correcting approximately for shrinkage of the vessels by a comparison of the length of the embryo at the time of fixation and after embedding and sectioning. It will suffice here to state the chief conclusions derived from the tables which were prepared from the measurements. In the further examination of the growth of the aortic arch it is to be remembered that there are three regions of the arch system to consider — the aortic trunk, the left half of the aortic sac, and the left fourth arch together with the paired aorta between it and the pulmonary arch (plate 2). These parts are to be compared, respectively, with the proximal end of the arch from valves to innominate artery (plate 3), the portion between the innominate and left common carotid, and the part between the left common carotid and the ductus arteriosus. The part of the left aorta which enters into the formation of the arch was not especially studied.
The distance from aortic valves to the left pulmonary arch, or, later, to the ductus arteriosus, which includes nearly all of the arch, does not increase from the late branchial period to the stage represented by a 24-mm. embryo with sternal bands in contact and the heart and large vessels in nearly their adult thoracic position. There is no reason for believing that this failure to elongate is only apparent and due to a proximal movement of the aortic ductus. If such a shifting should take place, it would naturally be greatest at the time of rapid descent, yet no change in the distance from the valves occurred at this time. Doubtless, then, there is a true standstill in longitudinal growth.
Though the arch does not elongate, it does increase in diameter. The measurements show that the left fourth arch and, to a less degree, the left paired aorta increase rapidly in circumference as the aortic arch is forming. The sac region of the
arch alone is much larger around in the post-branchial period than is the sac in the
branchial period. By these enlargements an arch is developed without local inequalities and with connection adequate to carry more than half of the entire current to
the dorsal aorta, which was formerly divided between six branchial aortic arches.
The changes in extent of the divisions of the arch will be best understood if the interval between the innominate and left common carotid be first considered. In the
early post-branchial period this is somewhat greater than the length of the left half
of the aortic sac, to which it was equivalent at the beginning of the period. It
reaches a maximum at about the time of the rapid descent of the arch (16 to 17 mm.
embryos) and decreases rapidly while the rudiments of ribs and sternum are closing
in to form the superior thoracic aperture. The increase in length indicates a real
growth, since the circumference of this region does not decrease, and it is evident
that the innominate and left common carotid rather precisely mark off territory
derived from the earlier left half of the sac during the first part of post-branchial
development and are withdrawing from each other at this time because the part of
the arch between their points of origin is conforming to the general body-growth.
The later approach of the two branches in embryos of 18 to 24 mm. length must be
due to a different process in the wall of the arch, for the increase in the circumference
at this time is not nearly as great as the decrease in distance between the two arteries. Hence we can not explain then* approach on the basis of a mere reshaping of
the wall of the arch between them by which it gains in circumference what it loses in
length ; there must have been an actual decrease in the substance of the wall of that
part of the arch or a plastic rearrangement, allowing the vessels to approach by one or both of them moving in a certain sense through its substance. As no good reason
for assuming that the arteries undergo a decrease of substance while maintaining
their diameter and function was found elsewhere in this study or in the literature,
this alternative may be dismissed from consideration, and it may be safely concluded that the substance of the wall has shifted about to permit a movement of the origin of one or both branches. The two arch divisions lying proximal and distal to the interval between the innominate and carotid arteries differ greatly in their changes in length. The segment proximal to the innominate, taken with the truncus aorticus, to which it is equivalent at the beginning of the post-branchial period, shows an increase in length which becomes very rapid when the innominate and carotid are approaching at the time of rapid descent. The part distal to the left common carotid, extending to the upper end of the sixth arch, shortens in the late branchial and early post-branchial periods and later remains constant during the time of rapid descent.
It is clear that in the history a sharp distinction must be drawn between the period before and the period occupied by the rapid descent. Before the descent the truncus arteriosus and the succeeding division of the arch which has developed from the left half of the sac increase in length. As has been seen, no marked increase in diameter is required, since in the branchial period these vessels are relatively capacious parts of the arch system. The distal portion of the definitive aortic arch coming from the left fourth arch and from the aorta distal to it is in contrast with the more proximal part of the forming arch. They remain unchanged in length up to the time of rapid descent. In circumference the part derived from the fourth arch undergoes an especially rapid enlargement, since in the branchial period it is only one of six conveyers of the blood from heart to aorta, while at this stage it transmits more than half of the entire current. At the time of rapid descent the innominate and the left common carotid approach, while the distance between the innominate and the aortic valves increases with especial rapidity. It is natural to conclude that the innominate has moved toward the left common carotid. Since the distance between the left common carotid and the ductus arteriosus remains constant, the former probably does not change its position on the arch. These inferences, drawn from the changes in length of the various parts, agree with expectations based upon the relation of the two vessels to the forming arch at the beginning of the postbranchial period. As the left carotid is at its summit and the innominate comes off from its ascending limb, only the innominate could respond to the tension upon it by moving along the wall of the descending arch.
A result of the retardation in the elongation of the distal part of the arch relative to the proximal is a change in the region which forms its summit. In the 14-mm. embryo, in which the arch is just taking form, the entire left fourth arch is the summit (plate 3, fig. 37). The relative shortening of the distal part of the definitive arch results in a drawing down of the fourth-arch zone into the descending limb, thus leaving the left common carotid at the summit (fig. 24). The distal migration of the innominate on the ascending limb also serves to bring it to a position on the highest part of the arch.
In its change of position the left subclavian involves both the arch and the aorta and helps one to understand the manner of their growth. The interval between the left subclavian and left common carotid, as also its approximate equivalent in the branchial period, shows a marked decrease not only relatively to body length but absolutely. In fact, it is only one-fifth as long in a 24-mm. embryo as in the late branchial phase. If we subtract from it the length of its proximal part as
far as the ductus arteriosus, it decreases to zero, since the subclavian shifts upon the aorta and arch upward past the ductus.
At its first appearance the subclavian arises from the unpaired aorta. It passes the bifurcation of the aorta early in its development and on to the left paired aorta. Its movement past the fusion point of the aortae and the ductus arteriosus can only be explained by a considerable shifting of the material of the wall of aorta and arch, and in this respect it resembles the changes in position of the innominate (figs. 18, 19, 22, 23). A similar condition has been found in the large abdominal arteries. Evans (1912) suggests that their movement along the dorsal aorta may be due to an unequal growth of the dorsal and ventral walls. The exact nature of the translocation of material which permits such shifting, however, seems to be at present very uncertain.
To summarize the observations on the growth of the definitive aortic arch during
the period of rapid descent of heart and arteries and the coming together of the
sternal bands, before the rapid descent the proximal part of the arch extending up to
the origin of the left common carotid elongates rapidly and increases moderately in
diameter. The more distal region, as far as the ductus arteriosus, decreases in
length. It increases rapidly in diameter, however, to compensate for its originally
small cross-section as compared with the more proximal parts. Increase in length
or diameter, if any, during the rapid descent, is too slight to be distinguished. The
chief changes are in the movement of the innominate and the subclavian along the
wall of the arch. The innominate moves up to the left common carotid, and the
subclavian approaches it from the other side. The subclavian passes the ductus
arteriosus but does not approach very close to the carotid at this time. The large
part of the arch extending down to the ductus arteriosus does not increase in length
during the considerable developmental interval included in this study, though its
diameter enlarges.
The history of the main post-branchial pulmonary channel illustrates the same
growth processes observed in the development of the arch. The first step is the
separation of the pulmonary trunk and its pair of arches from the aortic trunk
and sac (fig. 17a). Because the pulmonary arches arise from the sac close to the
mid-sagittal plane, little of the sac is removed when they separate off, and no
attempt will be made to trace the small zone derived from it in the later development. The proximal part of the right pulmonary arch remains as the origin of
the right primitive pulmonary artery after its distal portion degenerates. Relieved
of the longitudinal tension exerted by the complete arch, the angle between the
remaining part of the arch and the primitive pulmonary artery tends to straighten
out, aided, no doubt, by a formative action of the current not associated with
longitudinal tension, so that the boundary between the two can no longer be identified. The loss of this tension at the junction of the two pulmonary arches,
taken similarly with the action of the increasing current, permits the pulmonary
trunk and the left pulmonary arch to align (fig. 17, a to d). The resulting straight
vessel is the main pulmonary channel and carries the blood from the right ventricle
to the aortic arch until its distal end, the ductus arteriosus, becomes closed soon
after birth. The proximal segment of the right arch, now part of the right pulmonary artery, is still present to mark more or less definitely the zone corresponding
to the earlier point of origin of the pulmonary arches. An idea as to how long
the vessel mil serve this purpose may be obtained from the changes in dimensions
of the divisions of the pulmonary channel which it subtends.
There are three territories of the arch system to trace into the later pulmonary vessels: the pulmonary trunk from the valves to the origin of the pulmonary arches, the proximal part of the left arch up to the origin of the left primitive pulmonary artery, and the distal part of the arch from the artery to its upper end (plate 2). They are to be compared, respectively, with the later distance from the pulmonary to the origin of the right pulmonary artery, the interval between the origins of the two pulmonary arteries, and the length of the ductus arteriosus (plate 3).
The segment from valves to right pulmonary artery elongates during the
transition from branchial to post-branchial phase. It increases as rapidly as the
body length during the earlier part of the post-branchial period. The interval
between the two primitive pulmonary arteries remains for a time about equal to
the earlier segment of the left pulmonary arch up to the origin of the left pulmonary.
During the rapid descent, however, the two vessels approach, and before a length
of 40 mm. is attained they come off side by side. There is also no increase in the
length of the ductus arteriosus over the part of the left arch distal to the origin of its
pulmonary artery. From the late branchial period to the end of the period under
consideration the ductus decreases to one-fifth of its former extent relative to body
length.
The fact that there is an increase in length in the region of the main pulmonary
channel proximal to the two pulmonary arteries and a decrease in the portion distal
to them suggests the possibility that the points of origin of the two vessels shift
distally. At least while they are approaching each other, one or both of them must
move through the wall. However, a large part of the increase in the length of the
proximal division of the channel and the decrease of the ductus arteriosus occurs
before the distance between the two pulmonary arteries begins actually to decrease.
It is probable that at this time inequalities in longitudinal growth between these
two terminal segments are the chief if not the sole cause of the shifting of the
arteries. If this be true, in spite of the great decrease in length of the ductus
arteriosus relative to body length in the late branchial and the early post-branchial
periods, increase of its wall substance must still have been taking place, because in
this period its circumference is greatly augmented. By the rapid decrease in
relative length the ductus is approaching the small size, relative to adjacent parts,
which it maintains throughout its later existence.
The innommate and common carotid arteries change rapidly into long trunks as the aortic arch shifts caudally from the branches of the carotids in the head region. As was seen from the description of the 14-mm. embryo, the primitive innominate must, from its general relationships, be largely an elaboration of the right half of the aortic sac (plate 3, figs. 37, 39). It appears at this time as a transverse tube. As the arch makes its rapid descent, this swings around to a direction nearly parallel to the long axis of the body. Due to the rapid expansion of the arch the innominate takes on the appearance of a branch. Measurements show that its length remains about constant in the embryonic part of the post-branchial phase, but that at its beginning there is evidently a period of elongation, since it is longer than the right half of the sac. Its diameter equals that of the sac. In a series from the post-branchial period it extends over a distance of about one and a half vertebra; and is consequently much longer relatively than at maturity. The chief precursor of the external carotid artery at the end of the branchial period is found to be coming off from the third aortic arch near its origin. At the time when the arterial territories derived from the third and fourth arches can be distinguished only by means of the vanishing remnants of the aortae which lie between their upper ends it is found to have shifted out upon the third arch.
Kingsbury (1915a) has given a suggestive schema to show the influence of the widening metapharynx by the successive "moving out" of the first aortic arch upon the second, the second upon the thud, and the third upon the fourth. If we substitute the primitive external carotid for a persistent proximal end of the second arch and recognize that the aortic sac itself elongates rather than that the third arch moves out on the fourth, the schema is still useful as emphasizing the association of the lateral movement of ventral parts of the arch system and the vessels which succeed them with the lateral growth of the pharynx.
The portion of the third arch territory proximal to the primitive external carotid on either side constitutes the primitive common carotid artery. This vessel, like the innominate, elongates as the aortic arch moves away from the pharyngeal region and swings into a more longitudinal position (plate 2, figs. 35, 36; plate 3). As it passes upward, however, it still bends laterally and ventrally. This is a result of the large size of the head at this time relative to the neck. It is not possible to say with certainty whether the entire territory derived from the third arch is ultimately to be found in the common carotid. As this vessel elongates, it is possible that it also is pulled downward relative to the external carotid, so that the early shifting of the latter vessel, which we can recognize up to the middle of the third arch, may be continued the entire length of the arch, or even farther; or it may be that such a degree of elongation is effected by the growth of the region derived from the proximal half of the third arch that the external carotid does not shift beyond the middle of the region derived from the arch.
The late history of the right fourth aortic arch and the part of the right paired aorta caudal to it is bound up in the development of the right subclavian artery. The interruption of the left paired aorta cranial to the fourth arch and distal to the subclavian permits a swinging around of the arch and the remaining division of the aorta until they are aligned with the primitive subclavian (figs. 13 to 16). These changes will be more fully explained in the history subclavian arteries.
Topography of Aortic-Arch System and its Derivatives - Fusion of Primitive Aortae
The components of the branchial-arch system undergo shifting in the direction of all three axes of the body. Of these, the longitudinal are of greatest extent, while the dorsoventral are inconsiderable and are confined chiefly to movements of the arches which have already been discussed. In their movements the aortse are in several respects in contrast with the rest of the system and require separate consideration. The question of the lateral movements of the primitive paired aortse is bound up with their fusion and the two subjects will be discussed together.
It is not to be expected that the paired primitive aorta? and their continuations, the primitive internal carotid arteries, should maintain equal intervals between each other in all their parts throughout development, since they extend almost the full length of the body and must be exposed to many growth displacements by surrounding structures. There is the possibility that they may come into contact or that they may withdraw from each another, and in fact both conditions are realized in different regions. The more striking changes in the position of the arch system were appreciated by the early investigators. Von Baer (1828) pictures the caudal movement of the heart accompanied by a development of a ventral segment of the first arch and a caudal deflection of the ventral ends of the others. He also shows how the blood-stream is shifted by the loss of cranial and the appearance of caudal arches. His (1880) noted that the caudal ends of the third and fourth arches took on a more cranial direction at their proximal ends and described the changing direction of their arterial trunk. Tandler (1902) distinguished three of the various types of wandering: (1) of the " conus," causing a relative lengthening of the aortic arch; (2) upward displacement of the ventral portion of the arches; and (3) caudal shifting of the fourth and pulmonary arches. Kingsbury's analysis of the migration of the pouch derivatives and the related blood-vessels will be referred to later.
The primitive dorsal aortse during their earlier existence are separated from each other by a contact of nerve-tube, digestive tract, and notochord, which interpose between them a barrier of considerable width. This condition exists during the appearance of the earlier somites, but the nerve-tube and notochord gradually separate from the digestive tract, and mesenchyme moves in to fill the gap. Before long the two aorta? fuse in their intermediate portions which lie opposite the throacic segments. It was of interest to ascertain whether this is preceded by any actual approach of the vessels as a whole or whether only their adjacent walls draw near due to the increase in diameter of the vessels. A comparison on models was accordingly made between the interval separating the centers of the two vessels soon after their establishment and the corresponding distance in others in which the beginning of fusion was already indicated by the establishment of transverse communications. The distances divided by the magnification are for the earlier aortae 0.18, 0.16, 0.12, 0.15, and 0.12 millimeters; at the time of fusion they are 0.24, 0.15, 0.22, 0.14, 0.13, 0.22, and 0.24 millimeters. At the earlier time the average is 0.146 and at the later it is 0.177+ . Clearly, then, the aortas as a whole do not approach each other. If the slight difference in the average distance between
the earlier and later periods has any significance, it shows that the vessels are being
rallied slightly apart by the general growth of their surroundings. The models
show that at this time the rudiments of vertebra? and nerve-tube are expanding, so
that the aorta? are gradually taking on a medial position relative to their lateral
borders. Though the vessels as a whole do not approach, their increase in
caliber prepares for their fusion by approximating their adjacent walls.
The fusion in the aorta? occupies about a week. In its first stage the two aorta?
are connected by transverse anastomoses and lie almost in contact. No. 2053,
a 3-mm. embryo, is apparently the only recorded example of this condition in
man. It has 4 cross connections, the largest being of nearly aortic caliber.
Sabin (1917) figures a slightly more advanced condition in a 20-mm. pig embryo,
in which about 15 are present, some of the more caudal being of large dimensions.
Embryos No. 2053 to No. 2841, inclusive (table 1), all show the process of fusion
still under way, though a long, more caudal region of continuous fusion is already
present in each. Tortuous swollen capillaries or straight transverse channels of
larger dimensions connect the two vessels just cranial to the fused region. Enlargement of capillaries connecting the arteries and the development of larger transverse communications from them are clearly in progress. The process is comparable with the development elsewhere of vessels from a capillary plexus. At the
cranial end of the region of continuous fusion the unpaired aorta has the crosssection of a figure 8, often for a considerable distance. This evidently is the result of the recent blending of a series of transverse communications. Tracing caudally, a remodeling can be followed into a vessel of the usual form.
It has sometimes been assumed that the fusion of the aorta progresses cranially,
and the spinal ganglia or vertebral rudiments have been used as points of comparison. This method leads to entirely erroneous conclusions. During the time in
which fusion is taking place, the nerve-tube and cervical vertebral column are
growing cranially relative to the pharynx, to which the aorta is moored by its
arches. The relative position of the pharynx and these more dorsal structures
also shows much individual variability in the fixed embryo. Whether this occurs
in life was not determined. It is by a comparison with the immediate environment
of the aorta, especially the pharynx and digestive tube, upon which, for the time
being, it does not shift, that changes in the region of fusion may be recognized.
The most cranial communication, or, in the absence of a communication, the end
of the region of continuous fusion, is found in all but the youngest embryo, showing
fusion to vary in the branchial period from a position of 33^ to one of 5^ body
segments caudal to the pulmonary arch. For these measurements in embryos too
young to show the pulmonary arch, the position later to be occupied by the arch
was used in place of it and was recognized by the caudal pharyngeal-pouch complex.
In the youngest fusion stage the most cranial communication is 9 body segments
behind the sixth-arch region. The fusion is thus shown to begin more caudally
and progress forward. The presence of a region of continuous fusion caudal to
the territory where it is in progress in the next older embryos points to the same conclusion. A progress of fusion from an intermediate point forward and backward
is in fact to be anticipated from the relation of the primitive paired aortse just
previous to the beginning of the process, as they show a region of closer approximation from which they diverge both cranially and caudally.
A period of fixity in the position of the cranial point of fusion of the aorta?
relative to the pharynx begins with embryo No. 810 and indicates that fusion in a
cranial direction has been completed. The bifurcation remains stationary until
the aorta begins to shift caudally relative to the digestive tube and respiratory
tract. The cause of the arrest of fusion here is to be found in the active separation
of the vessels due to the pressure exerted by the expanding rudiments of the vertebrae and esophagus, between which they lie. As will be seen, a separation of
this nature is not unique for this region, but is much better marked in a more
cranial part of these vessels.
When fusion ceases, the bifurcation of the aorta is approximately opposite the seventh body segment. Relative to the nerve-tube, this point now lies more caudal than it did at an earlier period, due to the fact that a forward shifting of the cranial end of the nervous system relative to pharynx and aorta has been taking place more rapidly than the cranial progress of the point of fusion. In this way it comes about that in embryo No. 1075, for example, fusion, though still progressing, is opposite the second cervical ganglion, while in No. 810, in which the unpaired aorta is complete, it is opposite the seventh. In embryo Strahl 10, of the Keibel and Elze (1908) table, the aortic bifurcation is also given as opposite the second cervical ganglion.
The approximation of the walls of the primitive aortse in an intermediate region results in the existence of caudal paired aortse for a time after an unpaired aorta has become established. They are never long vessels, because, while they are extending caudally by their differentiation from a plexus, they are shortening at their cranial end by fusion. The paired condition, except possibly in the form of slender terminals, does not remain in this region, as at the cranial end of the embryo. In 4 and 6 mm. embryos only very short double vessels are present. In other embryos, ranging from 5 to 18 mm., the vessel is seen in section to be single, at least until it has shrunken to a very small caliber.
At the time the primitive aortse are fusing they are continuous with paired longitudinal neural arteries which pass backward under the brain. In the formation of the basilar portion of these, which is terminated cranially by the region of the hypophysis, there is a fusion much like that of the aorta. There is left a segment in the forebrain region which, like the cranial end of the paired aortse with which they are continuous, does not fuse. On the contrary, the two originally parallel vessels, each with its longitudinal neural and carotid parts, are carried away from each other to a greater or less degree in various regions, depending upon the activity of the lateral growth of the surrounding structures. In the late branchial period they have three well-marked regions of divergence. These reach their maximum opposite the middle cervical, the anterior pharyngeal, and the diencephalic regions,
respectively.
In the cervical region in a 12-mm. embryo the paired aortae lie in a little groove on either side at the plane of contact of the condensed mesenchyme of the vertebrae and the esophagus. It is apparently the expansion of these masses that has carried them apart In the anterior pharyngeal region the great widening at the level of the first and second pouches has carried the aorta with it just as it has carried apart the first and second aortic arches on the ventral side of the pharynx. In the early part of the branchial period the first and second arches probably aid in the separation by holding the aortae close to the lateral borders of the pharynx as it widens. The most cranial divergence of the paired vessels is in the territory of the longitudinal neurals and is the result of the growth of the forebrain, upon which they lie. On plate 2 (figs. 33 and 35) are shown the caudal and intermediate curves.
The regions of approximation of the aortae are interesting, since they must correspond to the territories of sluggish lateral growth in the environment. The more caudal of these is at the esophageal end of the pharynx, and therefore includes the attachnment of the fourth and pulmonary arteries. It is not surprising that growth should be slight here, since this division of the pharynx, as is well known, shows many regressive features. It is of interest that it has not only affected the course of .. (--Mark Hill 17:32, 7 May 2011 (EST) text illegible).. the post-branchial period the vagus nerve often leaves an impression on the aortae as it curves around their outer surface in its caudal and ventral course to lungs and digestive tract. It may be that the nerve exerts a minor influence in maintaining a close approximation of the two vessels.
The proximity of the arteries just in front of the pharynx indicates that the mesenchyme here has not expanded laterally as fast as the pharynx behind and the forebrain in front. There has been some separation of the vessels such as one would expect as an expression of the tendency of any growing vessel to straighten its tortuosities through the action of hydrodynamic factors. It may be that failure of the artery to grow as fast as the nerve-tube and pharynx may have assisted in decreasing the curvature engendered by the longitudinal tension.
The last important lateral displacement of the aorta is the movement of the
entire thoracic aorta from the mid-line to a position more or less completely over to
the left surface of the vertebral body. A lateral shifting at the bifurcation begins to
show itself as soon as the right paired aorta has begun to decrease in volume relative
to the opposite vessel. In a 50-mm. fetus we find the aorta and the esophagus both
in contact with the vertebra in the thoracic region and lying to either side of the
mid-sagittal plane. While the lateral movement at its beginning is, to a certain
degree, a mere straightening of the angle between the left paired and the unpaired
aortae, due to hydrodynamic forces or longitudinal tension resulting from inequality
in growth between the aorta and its surroundings, most of the displacement is
doubtless the result of pressure from the vertebral column above and esophagus
below. It is the same process which already has been found to cause the separation
of the paired aortae in the region just cranial and is doubtless due to the same causes.
It is probable that the initial deflection of its upper end, due to the retention of
the left instead of the right paired aorta, is the cause of its slipping to the left rather
than the right. It is of interest in this connection that Krause (1868) , in his discussion
of arterial anomalies, states that the retention of the right paired aorta and right
arch is frequently accompanied by a dextral position of the thoracic aorta.
The changes in position of the aorta along the transverse axis may be classified,
therefore, as of three kinds: (1) a further separation in the middle cervical and
anterior pharyngeal regions, with which may be grouped a separation of the paired
longitudinal neural arteries under the forebrain ; (2) an approximation of contiguous
surfaces due to growth of the vessels in caliber, chiefly in the thoracic region, which
results in their fusion by means of anastomoses; (3) a translocation of the thoracic
and abdominal aorta? toward the left side of the vertebral column, due to the
pressure of structures lying dorsal and ventral to it.
Migration of Aortic-Arch System - Longitudinal Shifting of Aorta
It would be easy to interpret the cranial elongation of the region of fusion of the aorta? as a cranial shifting of the unpaired vessels did not the presence of transverse communications and peculiarities in the form of the cranial end of the fused region point to its true nature. The true caudal shifting of the aorta? begins before fusion is complete; yet there is no reason for confusion of the two processes, since it is only the cranial end of the paired vessels that is at this time involved.
The moving of the aorta relative to its surroundings is progressive, beginning
in the region of the first aortic arch, perhaps even farther forward, and gradually
extending to more cranial parts of the vessel. There can be no doubt that it is due
to a slowing down of the longitudinal growth relative to the pharynx and digestive
tube, and this must first take place only at the cranial end, later manifesting
itself in regions progressively more caudal.
The first indication of the caudal movement is the shifting of the third aortic
arch from a position at the middle of its visceral arch to its most caudal border and
the bending backward of its upper end before entering the aorta (plate 2, fig. 34).
These changes are in turn followed by the other arches, until, in the late postbranchial periou (plate 2, fig. 36), even the pulmonary arch, as we have seen,
bends markedly backward at its upper end before entering the aorta. At this
time, also, the shifting can be seen, by the sharp caudal bend of the proximal end
of the more cranial cervical segmentals, to have proceeded beyond the pharynx
(fig. 27) . The more moderate cranial slope of the distal part of each of these arteries
is due to another cause, namely, the shifting of the nerve-tube relative to the
digestive tract, which forces an oblique direction not only on the part of these
vessels but also on other structures of the body segments lying between them.
The aortic region involved in the shifting does not extend to the bifurcation
until the end of the branchial period. There is therefore a considerable interval
of time, beginning with the completion of the pulmonary arches and extending to the involution of the right pulmonary arch, in which the bifurcation is at rest.
As has been said, it lies at this time opposite the seventh body segment. In the
early branchial period, as the arches are freed for further backward progress by
the caudal shifting of the derivatives of the pharyngeal pouches and the successive
interruption of various parts of the arch system, the cranial portion of the unpaired
aorta itself moves caudally relative to the adjacent digestive and respiratory
organs and the more distant organs as well. This is best shown by the movement
of the aortic bifurcation.
The paired aorta is followed in its descent by the left unpaired aorta only.
The right, fixed by its subclavian branch, gives way in a short terminal segment
between subclavian and bifurcation in a manner previously described. The
process is just beginning in one 16-mm. embryo of our series, while in another of
the same length the segment has stretched to a thread whose caudal termination
shows the point of bifurcation to have descended from a region opposite the sixth
cervical to the second thoracic vertebra (fig. 14). Thyng (1914) also finds it
here in a 17-mm. embryo. It is probable that a rather common type of anomalous
subclavian described in the adult indicates roughly by its origin the ultimate
position of the region corresponding to the former bifurcation. It is characteristic
of these anomalous vessels that they pass between vertebral column and esophagus
and come off as the most distal branch of the arch, if, indeed, they do not arise
from the descending aorta itself. Their existence is probably due to the fact that
in their development they tap the main stream through the caudal end of the left
paired aorta instead of making use of the right aorta and the fourth arch. Subclavian of this kind are found in the adult arising from the termination of the
arch or the aorta as far caudal as the fifth thoracic vertebra. Since the subclavian
and other branches of the arch shift cranially upon it, there is a possibility that the
aortic wall derived from the earlier region of bifurcation lies still lower. Granted
that the region of bifurcation in the adult lies at the sixth thoracic, the distance
at this time between it and the ligamentum arteriosum, which succeeds the arterial
duct, can not be more than the length of 3 body segments. In the branchial period
the bifurcation lies about 5 segments behind the pulmonary arch, as determined
on models of 10 embryos. There is, then, during development, a relative shortening
of the part of the definitive aorta derived from the left paired aorta, Since it has
been found that the distal part of the aortic arch, and probably also the distal part
of the main pulmonary channel, lags in growth behind the proximal part during
the early post-branchial period, it can now be said that the proximal part is in
contrast to the aorta as well as to the distal part. This contrast in growth in the
different parts of the chief arterial trunks leading from the heart is an interesting
condition. Perhaps it should be regarded as illustrating an accelerating effect
of increased longitudinal tension upon the growth of the arteries due to the descent
of the heart.
It is well established that the caudal end of the aorta withdraws cranially.
Since the two ends approach each other, there must be a region not far from the
thoracico-abdominal boundary where there is little shifting in either direction.
No connected account of the shifting of the dorsal aorta is to be found in the
literature. Goette (1875) has noted in the toad the retreat of the bifurcation
point of the aorta, and Hochstetter (1890) finds it to be a regular occurrence in
amniote development. Both observers fall into the error of regarding it as the
result of splitting. Hochstetter further states that it results in the lengthening
of the aortic roots, which term he applies to the paired aortse caudal to the pulmonary arch. In man, at least, as has just been seen, their change in length is
of the opposite kind.
Shifting of Arches and their Ventral Connections
The movement of the dorsal aortse is but part of the general descent of the cervical viscera into or towards the thorax. The heart and aortic arches not only share in the movement but are not exceeded by any other structure in the distance covered. The shifting of the aortic sac in the branchial period is slow and corresponds in amount to the aortic displacement at this time.
The sac moves backward along the floor of the pharynx, keeping pace with the
appearance of new caudal aortic arches and the disappearance of the more cranial
ones. Kingsbury shows that the apparent distance it has moved is enhanced
by the active forward growth of the anterior pharyngeal region. It is not clear
whether the movement of the sac at this time is a translocation of the entire structure relative to the pharynx or a mere growth backward of its caudal portion by
the development of successive bays which take part in the formation of the arches
as they appear one after another. The constant position of the trunk relative
to the sac speaks for the former view. In the post-branchial period there can be
no doubt of a translocation of the sac. The extent of this journey may be learned
from the succeeding account of the migration of the fourth arch, since the two
move approximately the same distance.
The movement of the more caudal aortic arches through their visceral arches
has already been referred to. At the end of the branchial phase of development
the arches present at that time are hooked around the structures which are apparently preventing their caudal progress. The pharyngeal pouches are in the way of
the two more cranial arches, while it is the vagus and recurrent nerves which seem
to bar the way of the pulmonary arches. After their development from the pouches the pharyngeal derivatives lose their connection with the pharynx, thus removing the obstacle to the migration of the third and fourth arches. The right pulmonary arch, being under less favorable conditions of current-flow than its mate, undergoes degeneration. The left persists and apparently forces the recurrent nerve of its side to elongate in order to give way to its advance.
Sufficient for an illustration of the shifting of the arches is the left fourth arch, which gives rise to a zone of the definitive arch. At its first appearance it is below the first occipital segment, while in the adult the definitive arch overlaps the second and third vertebra, and the zone of the fourth is caudal to the summit of the arch. The fourth arch and its derivative tissue therefore shift the length of 13 body segments (figs. 22 to 25), but not nearly so far in relation to the immediate environment (the pharynx and digestive tube), for they are only about 43 body segments apart when the arch reaches its adult position. The movement of the fourth arch and later its derivative territory in the definitive aortic arch is very rapid after the arches are freed from the pouches and vagus nerve, though somewhat slower than the descent of the cranial end of the aorta at this time. Between the developmental stages represented usually by embryos 14 and 18 mm. long it has moved the length of about 2 vertebrse, at the rate of about one-fourth of a vertebra a day.
The heart bears a changing relation to the sac and arches. In the first-arch stage the axis of the arterial end of the heart is dorsoventral as it reaches the base of the arches. Some of the truncus arteriosus is seen to approach the arches from their cranial side (plate 1, figs. 30 to 32). This condition persists until near the end of the branchial period, accompanied by great fluctuations of the proximal end of the truncus to right or left in different individuals, as also noted in the chicks by Kranichfeld (1914). In the succeeding period the trunk is reversed so as to approach the arch from the caudal side (plate 3, fig. 37). At the time the change takes place the division of these into aortic and pulmonary parts has permitted wide separation of the lower ends of the fourth and sixth arches (plate 2, fig. 36). The reason for this condition is not clear, but it is possible that as the long axis of the heart swings past the perpendicular the heart may crowd the pulmonary and aortic trunks, thus pushing them apart.
The ultimate reason for the movement of the aorta and arches, judged from the standpoint of individual development, is the same as already given for the retreat of the cranial portion of the aorta; in each instance it is the unequal growth of different organs or regions. The cranial expansion of the forward portion of the central nervous system and skeleton surpasses that of the aorta, the heart, and ascending part of the arch, together with certain structures lying caudal to them.
In following the breaking down and movements of the parts of the arch system various arterial changes have been described that were apparently in part due to a longitudinal pull produced by the descent of the heart and the aorta. It will be well to summarize these in order to better evaluate the influence of this factor on arterial growth.
The subclavians are forced to move along the arch, and the innominate and common carotids are swung around into a more longitudinal position. As their points of origin recede, the latter elongate rapidly, as do also the proximal portion of the aortic arch and probably the corresponding portion of the early post-branchial pulmonary channel. At an early stage in the descent, segmental arteries and 3 segments of the paired aorta? contract, atrophy, stretch into long filaments, and finally give way.
The complications resulting from the lateral movement of the fourth and sixth arches, due to their continuity with the aortic sac and the aorta, are interesting. At the beginning of the post-branchial period the two pairs of arteries are bent around the pouch complex and the vagus nerve, respectively, as though the heart and aorta were pulling them caudally against these structures. Later, we have a contrast between the history of the right and left fourth and pulmonary arches, apparently because in each instance the leftvessel is now receiving a larger current, and thus can react more vigorously toward its environment than its counterpart on the right. Thus the left fourth moves caudally faster- than the right. The left pulmonary, though sharing the pressure of the vagus in its caudal surface, does not undergo involution but is able to move caudally. Apparently it forces the vagus and recurrens before it. Certainly they do elongate the loop which held it so that it can descend to its ultimate position. Most striking of all is the caudal shifting of various vessels, as, for example, the definitive arch.
Among these various apparent effects of pull exerted by the descending heart and other structures, some, as, for example, the stretching out of vessels into filaments at a late stage of involution, are so obviously due to this cause that a discussion is unnecessary. In the early stage of interruption of the arches and the shifting of the arterial branches on their main stems the action of pull is difficult to establish with finality. Experimental evidence or its equivalent (the study of anomalies) is needed. In the sidewise progression of the definitive arch and pulmonary channel there must certainly be factors involved other than the caudal pull at their ends; yet there can be little doubt that in all of the arterial transformations the pull of the heart and shifting of the dorsal aorta are important factors.
A demonstration of the interplay of longitudinal tension of different confluent vessels has been seen each time a segment of the arch system gave way, and in these instances some of the arteries were showing merely the tension proper to them and entirely independent of a pull due to growth displacements. When one of three converging segments of the system underwent involution and its longitudinal tension weakened, the pull of the other two segments overbalanced it, thus stretching it and straightening the angle they formed with one another. This process indicates that under usual conditions the pull of any two such vessels counterbalances the tension exerted by the third.
A helpful analysis of the movement of the structures of the neck down to and into the thorax has been given by Kingsbury in his study of pharyngeal development. He describes their displacement to fill the space left vacant by the descending heart as a "growth eddy." He points out the complexity of the forces affecting the caudal shifting of the pharyngeal derivatives and expresses his belief that the mesenchyme also moves downward. This is a very appropriate characterization of the movement in its most salient features. It implies, however, a passivity of the structures coming in to occupy the space which probably is not the exclusive condition in any one of them. The arteries seem to act rather vigorously upon their surroundings during then descent. This is indicated by the differences in the relation of the right and left pulmonary arches to the vagus and recurrens nerves which have just been described. The fourth arch also gives evidence of helping to move the structures which earlier barred its way. In the 14-mm. embryo a pharyngeal-pouch derivative is found on each side, lying in contact with the caudal surface of the fourth arch, although one of them has moved a vertebra length more caudally. The larger and more rapidly moving arch has apparently aided in the movement of the pharyngeal tissue which lies in its path. Kingsbury has pointed out that the shifting of even the pharyngeal derivatives is in part due to their own outgrowth.
In a succeeding discussion, based on models prepared to show the rudimentary ribs and sternal bands as well as arteries, it wall be seen that the changes in the ribs are also somewhat suggestive of material in the growth eddy.
Relation of Migrating Arch and its Branches to Superior Aperture of Thorax
The approach and entrance of the heart and its arterial vessels into the thorax is characterized by a nice coordination between the time of arrival of the heart and aortic arch at the thorax and the coming together of the ribs and sternum in front. Within an interval of 10 days the upper ribs on each side, capped by their sternal bands, have completed the thoracic arch (figs. 18 to 25).
Figs. 18 to 25. The descent of the fourth aortic arch and the definitive aortic arch into the thorax, shown in relation to the cervical vertebrae and ribs. Asterisk, so-called fifth aortic arch; 4, fourth aortic arch; d. a., definitive aortic arch; R. 1, first rib; in. art., innominate artery; c. r., cervical rib; c. c, common carotid; v. art., vertebral artery; a. r., remnant of segment of dorsal aorta, interrupted between third and fourth aortic arches; sub. art., subclavian artery. In figures 20 and 24 the sternal bands are not yet in contact above and the definitive aortic arch has a large radius of curvature. In figures 21 and 25 the bands have met and the arch has become sharply bent by the swinging dorsally of the heart.
In the 14-mm. embryo the ribs are slightly concave cranially and a nearly straight in the transverse plane. By the time the embryo is 24-mm. long they have grown forward and around so that the sternal bands as capping their tips are fusing in the mid-line. Three models between the earliest and latest stages of this series show the rib as a whole sloping cranio-ventrally, but at 24 mm they are once more horizontal. Between the earliest and latest stages the rib elongates about threefold. It grows forward and medially, reshapes itself, and expands. It gives the appearance of passively swinging around to fill the space left vacant by the heart, and this effect is enhanced by its free end swinging downward as well as forward. There is, however, as above indicated, an expansion and a reshaping of its substance, not a participation in a passive eddy movement. It is not improbable that the mechanical influences of the descending heart, which cause the other structures to migrate, have a formative effect upon the growth of the ribs.
Since the upper ribs move in with other structures to fill the gap left vacant by
the heart, the close correlation in time between descent of the heart and closure
of the upper thoracic wall becomes understandable. Arterial arrangements which
would have tended to crowd the superior thoracic aperture are gradually altered
as the heart sinks into the thorax. The movement of the innominate and the
left subclavian near to the summit of the aortic arch is of this nature. There is
also the bending of the arch so that its dorsoventral diameter is decreased. Most
important of all are the changes in the position of the heart. At the beginning of
the period, in the 14-mm. embryo, the direction of aortic and pulmonary trunks
indicates that the apex of the heart is pointed well forward and that much of its
bulk lies ventral to the tip of the ribs. In a 20-mm. embryo the superior thoracic
aperture has become closed, and in correlation with this the definitive arch has sunk
below the level of the aperture and the heart has swung upon it as a hinge, so that
it points more caudally. To have arrived at this position, the apex of the heart
must, within a week, have not only moved with the arch at the rate of half a body
segment a day but, because of its caudal swing, must have exceeded the arch considerably in speed.
Individual Arteries
Pulmonary Artery
In tracing the development of a blood-vessel its history remains incomplete until one recognizes not only the capillary plexus from which it is derived but also the source of the angiobla^tic mass giving rise to the capillaries in the event they do not arise, directly from an open vessel. In the case of the primitive pulmonary arteries of higher vertebrates, which later evolve into approximately the right and left branches of the definitive pulmonary artery, the manner of origin of the angioblastic material seems to be well established. Fedorow (1910) and Bremer (1912) trace it in the rabbit and guinea-pig to paired growths from the aortic sac which they believe grow out to form a net from which the pulmonary arch is in turn derived. These authors figure reconstructions of the net. Buell (1922), in the most recent contribution to the development of the pulmonary vessels, which appears in this volume, also traces the angioblastic material in the chick to this source. Huntington (1919) is not in agreement with these observers, as he derives it in the cat from the dorsal aorta. The method used by him in making his preparations is not clear, and the formations figured are too unlike the findings of other writers to constitute satisfactory evidence in support of such a contention. There is also disagreement as to the form and position of the earliest pulmonary vessels themselves. Bremer and Fedorow find that a slender artery first extends caudally from the aortic sac and then becomes connected midway with a channel to the dorsal aorta. The free end of this vessel on either side constitutes the primitive pulmonary artery; the proximal part, together with the connection with the dorsal aorta, forms the pulmonary arch. Buell's injections of the chick show that the primitive pulmonary artery arises in this form not from the ventral-arch sprout but from the aortic sac, and that it is secondarily carried up upon the arch by the rapid development of the ventral end of the arch. Huntington describes in the cat the development of an isolated channel from the pulmonary plexus which is later tapped by a short outgrowth from the pulmonary arch to form each primitive artery.
The observations to be given on the development of the human primitive pulmonary arteries were made for the early stages from the study of cross-sections alone and in larger embryos by the preparation of models.
Embryos in which the vessels are well distended show that the earliest pulmonary plexus is already present at the time of establishment of the fourth arch. At this time the endodermic lung-bud is connected with the esophagus for most of its length. A net of large capillaries and of angioblastic cords extends backward from the aortic sac under the caudal pharyngeal-pouch complex and for a short distance up its posterior surface along the course of the later pulmonary arch. From its caudal extremity this plexus also sends a less developed net a little distance along the under surface and side of the laryngeal rudiment and common tracheo-esophageal mass. At this time large capillaries can be seen extending down as a plexus from the aorta into the esophagus. Later, there is a continuous tracheo-esophageal net of uniform character due to the meeting of the two earlier territories.
The earliest primitive pulmonary artery that could be recognized with certainty by a study of cross-sections was in an embryo with well-distended vessels
soon after the completion of the fourth aortic arch. It could perhaps be demonstrated still earlier by total injections. At this time the lung-bud was of considerable length and the primary lobes well elongated. A slightty later stage is shown
on plate 2, figures 33 and 34.
A search was made for an arterial rudiment in the tracheo-esophageal groove independent of the pulmonary arterial outgrowth from the aortic sac, such as Huntington believes to exist in the cat. The result was entirely negative, though 6 embryos of the proper stage in excellent state of preservation and with moderate vascular distention were examined. The region showed no vessel of greater than capillary caliber until the extension from the sac-vessel had reached into it. Some rather larger endothelial tubes were found on the dorsal surface of the lung-bud bifurcation, even before the fourth aortic arch was complete, but when followed in then development they proved to be the rudiments of the vein shown in figures 33 and 34. Buell has seen this earlier in his chick injections and terms it the "cephalic pulmonary tributary." He finds it to be a transitory vessel.
The succeeding history of the primitive arteries is connected with the transformations of the pulmonary arch. The study of our series led to the same conclusions as held by Bremer and Fedorow as to the developmental relations of the
two arterial channels. Embryos in good histological condition showed the presence
of a pair of arteries extending backward from the aortic sac before the pulmonary
arch was complete (figs. 33 and 34). The arch was formed by the establishment
of a connection between this vessel and the dorsal aorta in the plexus lying caudal
to the caudal pharyngeal complex. Just after the arch has completed its channel,
its divisions proximal and distal to the pulmonary artery join at a marked angle.
As the arch increases in caliber this disappears in a continuous curve.
The next important change in the pulmonary arterial vessels is the interruption of the right arch which has been already described. As the distal portion of
the arch degenerates, the angle between its proximal division and the right primitive pulmonary artery is gradually lost, and the segment from now on functions
exclusively as the proximal end of the artery (fig. 17, c, d). The mechanism of
these changes has already been sufficiently discussed. The interruption of the
arch also similarly allows a straightening of the angle at the junction of the pulmonary trunk and the left arch. Since the origin of the right pulmonary is at the
plane where these two territories are confluent, it is carried to the left, and the
artery is made to pass obliquely across the trachea and also to sink ventrally, so
that it is forced to curve slightly around the ascending limb of the aortic arch.
In the further history of the pulmonaries we must distinguish clearly between
the earlier post-branchial phase and the period of rapid descent of heart and large
vessels. Measurements on models of a ^orics of 11 embryos showed that the
distance between the origin of the two vessels remained nearly constant and equal
to its precursor (the proximal segment of the left pulmonary arch) until the period
of rapid descent. Then there was a quick approach, so that in a 24-mm. embryo,
with the upper part of the sternal bands fused, the two vessels were almost together.
They were found in contact in fetuses of less than 40 mm. in length (fig. 17, e).
The rapid approximation of the vessels and their final meeting can not be explained
by the slowing ingrowth of the wall between them. As in the movement of the
innominate and subclavian, there must have been actual progression of the vessels
at their origins through the substance of the wall of the parent vessel.
Bremer (1902, 1909) has made an interesting suggestion as to the nature of
the approach of the two pulmonary arteries, based on the observations at later
stages in the formation of the adult pulmonary artery and its branches in a number
of mammals. He believes that the pulmonary stem undergoes torsion, and that
the approach of the two arteries is due to then* fusing with it as a result of their
proximal ends being wrapped around it. In his second article on the subject,
referring to man, rabbit, sheep, and cow, he says:
- "With the growth of the truncus pulmonalis and its torsion about the bulbus aorta?
the two pulmonary arches are wound, as it were, around the bulbus and their walls brought into contact are absorbed so that the truncus pulmonalis grows longer at their expense, the point of bifurcation moving continually further from the heart. The left arch being the outside one in this rolling-up process receives the most pull, becomes the straighter and therefore the larger vessel and is shortened more rapidly. As a result the truncus pulmonalis reaches the left pulmonary artery while the right is still seen arising from the right arch some distance dorsal to this point."
It can be seen from the described changes in the relative length of the parts
of the main pulmonary channel incident upon the formation of the ductus arteriosus, that the interval marked off by the two pulmonary vessels moves distally
on the pulmonary channel. This is as one would expect if there were such a wrapping of the pulmonary arteries around it as Bremer describes. As an argument for
rotation and wrapping, however, this last circumstance loses much of its force
when it is recalled that the segment on the aortic arch between innominate and
left common carotid also moves distally on the arch as it grows shorter. Yet there
is no reappearance of rotation of the arch nor has such been claimed. The relations
of the arteries to the parent stem are not what one would expect were they brought
into contact with it and fused as a result of its rotation. In the period preliminary
to the rapid descent the primitive pulmonary artery comes off ventro-medially
from the stem instead of from the right side, as one might expect from the source
of this part of the vessel. The left pulmonary has retained its primitive ventromedial origin. After the descent the two vessels come off a little more ventrally.
This change in the position is of a kind that might have been caused by a slight torsion of the stem. To produce wTapping, however, they would have to be carried
around much farther to the left. One wxrnld also expect their right side to be bent
over against the main stem. Instead of this, the two arteries are nearly radial to
its cross-section (fig. 17 d).
We do not know whether the movement of the two pulmonary arteries taken
together is due to the shifting in the wall of the pulmonary stem or whether it
results from a retardation in the growth of the pulmonary channel distal to them,
as was earlier pointed out. The approach of the two vessels is certainly due to a
movement of the origin of one or both through the substance of the wall of the
main pulmonary stem. It is probable that longitudinal tension of the arteries
exerts an important influence in this process, and this, perhaps, may be caused by
the elongation of the lung rudiments. It is of interest that the curve of the right
pulmonary around the aortic stem is straightened out by the time the two pulmonaries have met, and it may be that the approach of the origin of the two vessels
is entirely due to the shifting distally of the origin of this vessel in the course
of its straightening.
By the coming together of the two primitive pulmonary arteries the organization of the pulmonary vessels at the end of prenatal development is closely approached. There is a pulmonary artery giving off a right and a left branch and a ductus arteriosus connecting the latter with the aorta. The pulmonary artery is formed from material derived from the pulmonary trunk and more or less of the left pulmonary arch of the branchial period. The arterial duct owes its origin, in large part at least, to material developed from the distal part of this arch. The two pulmonary arteries are morphologically dissimilar to the extent that the right has a zone produced from the proximal end of the right pulmonary arch, while the left has no corresponding region derived from an arch.
Subclavian Artery
The development of the subclavian artery in its early stages is a convincing illustration of the capacity of the blood-stream to take over and remold into a unit various vascular channels as the need arises. Though it shows great plasticity and inconstancy at this time, yet later, when it is more mature, it meets with surprising success in maintaining itself when exposed to stress due to the shifting of the surrounding organs upon one another.
In the development of the early arterial supply to the limb-bud there are considerable differences between the bird and the mammal, although at such an early
period one might with reason anticipate a close correspondence. Rabl (1907),
who has studied the condition in the duck by graphic reconstructions, describes a
period in which there is an increase in the number of small arteries lying in successive intersomitic spaces and passing from the aorta to the plexus of the early limbbud. Evans (1909a) confirms this in the chick by injections, and figures as many as
four vessels passing to the bud plexus along the planes of separation of the body
segments. He finds that at a still earlier period, before the 34-somite stage, there
are already distinct arteries of supply to the plexus from the aorta, but they are
not segmental. The vessels, both of the non-segmental and the segmental types,
are referred to as subclavians, but most of them have so little to do with the development of that artery that it will be best to designate them merely as limb-bud
arteries.
In the human embryo, Keibel (Keibel and Elze, 1908) described two limb-bud
arteries from successive segments at the time of the first appearance of the vessel.
Evans (1908) also found a similar case, though not from the same two segments.
Goppert (1909) figures, from graphic reconstructions of the mouse embryo, both
segmental and non-segmental limb-bud arteries. He also claims that the segmental type can be traced as independent channels well within the bud and discusses their changes. Since a segmental arrangement here implies a segmentation
of the substance of the limb, there is little probability that he is correct. Woollard,
in this volume of the Contributions to Embryology, gives very complete illustrations
of both arterial and venous vessels of the early limb-bud from injections of pigembryos. He does not find, at any time, more than one distinct artery to the limbbud. It is a branch of a segmental or, more precisely, intersegmental artery and
passes to the limb through the intersegmental space. There are short twig-like
vessels in other intersegmental spaces which come off from the segmental artery
and quickly go over into a plexus lying largely in the intersegmental spaces and connecting them with the limb-bud plexus. As the embryo enlarges, these secondary
connections do not increase in proportion to the single limb-bud or primitive subclavian artery and soon become negligible. Woollard does not find the nonsegmental limb-bud arteries of Goppert. His study throws doubt upon the existence of both multiple segmental and earlier non-segmental limb-bud arteries in
mammals, though the possibility of a certain amount of individuality in this regard
in the different species must not be lost sight of.
The earliest vessels to the limb-buds that I was able to recognize in the human
embryo (4 to 6 mm. in length) were found in specimens in which the fourth arch
was present but the sixth was not complete. The anterior limb-buds were as yet
but slight elevations from the general body-wall ; they had not been penetrated by
the outgrowing nerves, and they contained a nearly homogeneous vascular plexus.
One definite artery was present for each forelimb bud, and this lay in an intersegmental space. Enlarged channels could frequently be traced a greater or less
distance from the segmental arteries in other intersegmental spaces toward the
plexus of the limb-bud. Their appearance in sections favored the interpretation
that they usually broke up into a plexus at a greater or less distance from the limbbud and were thus similar to the twigs described by Woollard. It seems not unlikely, in some instances, that there were also one or more secondary channels
traceable as definite vessels to the plexus, though it was not possible to prove this
from the study of sections on account of their small, almost capillary caliber. The
second subclavians in human embryos described by Keibel and Evans are probably
of a similar nature, but the existence of such vessels can be proved only in sections
cut very favorably and probablj ~ T '>11 not be established without the use of in toto
preparations of complete injections.
In slightly older embryos it was impossible to trace the primitive subclavian
into the limb itself where it continued as the primitive brachial artery. At this
time the subclavian is found to be coming off from an outpocketing of the aorta,
which at the same time gives rise to a dorsal segmental branch. Later, this connection elongates into a definite vessel (the stem of the primitive segmental artery)
in the manner described by Rabl (1907) and Sabin (1917) in other forms.
In a model of a 5-mm. embryo the advance in differentiation of the limb-bud
is marked by the entrance of the spinal nerves into its base and is reflected in the
vascular system. A venous marginal sinus draining into the umbilical vein, as
described by Evans (1909a, 19096) in the pig and chick, is now well defined. The
segmental branches of the post-caval vein can be followed between the spinal nerves
to their origin in the brachial plexus. They are accompanied by branches of the
segmental arteries. The primitive subclavian is a branch of the seventh cervical
segmental artery. Within the body it lies between the sixth and seventh nerves
and passes over the dorsal surface of the plexus and soon breaks up into capillaries.
The model of the limb-bud of a 7-mm. embryo shows the limb considerably
elongated and containing an axially placed nerve mass which is already giving off
branches. The primitive subclavian has now become surrounded by the brachial
plexus. This seems to be due to the growth o neurons across its dorsal surface
to complete a canal about it, not to any development of a new arterial channel
through the plexus. The brachial artery is divided into three terminals. At
14 mm. the primary branches of the nerves and arteries are well developed in the
arm. Not only the radial, ulnar, and interosseus are present, but digital branches
as well. Their development was not followed.
The model of a 5-mm. embryo, in which a primitive subclavian was well
developed, showed a slender channel passing from subclavian to brachial artery
over the dorsal surface of the plexus. Goppert (1909) explained various loops passing around and through the plexus as resulting from anastomoses between a series
of limb-bud arteries and their brachial continuations in the limb-bud. Since
the evidence of a limb-bud artery in more than one space is the exception in man
and was not found to occur in the pig, we must regard such accessory channels as
the result of a chance enlargement of a part of the general plexus.
At the beginning of the post-branchial period the primitive subclavian has
thoroughly incorporated the territory derived from the stem of the earlier segmental
from which it arose, and thus the latter has entirely lost its identity (figs. 11, 12).
The serial position of this vessel can at first be told by its relation to the body segments. After its identity is lost in the subclavian, its place in the segmental series
may be inferred from the vertebra into which the vertebral artery enters. A series
of 15 embryos were examined to learn how constantly it arises from the seventh
cervical segmental. The specimens ranged from 4 to 24 millimeters in length, and
in the youngest the fourth arch had just been completed. In 2 of the younger ones
the subclavian comes off from the sixth cervical segmental artery, while in the
others it comes off from the seventh. In 1 embryo of the post-branchial period
both vertebrals enter the transverse foramen of the rudimentary fifth cervical
vertebra (plate 3, figs. 37 to 39) ; in another the right vertebral enters the fifth and
theleft the sixth. In the other 4, both vertebrals enter the sixth transverse process.
The frequency of variation from the origin of the primitive subclavian from the
seventh segmental noted here and of the corresponding entrance of the vertebral
into the sixth vertebra is far greater than is encountered in adult life. Since 2 of
the embryos were in a very early limb-bud stage, when its supply is scarcely more
than a plexus, it may be that, had death not occurred, a readjustment might have
soon taken place by the enlargement of a twig in the interspace usually occupied
by the seventh segmental superseding the aberrant subclavian. In 1 of these
embryos there was an artery extending nearly to the limb-bud in the usual position.
Whether or not this is the true explanation of the occurrence of these early aberrant
vessels, the other embryo, which is in the early post-branchial phase with the
vertebral already formed, was apparently in the course of a return to a usual type
in an entirely different manner. The vertebral has a double connection with the
subclavian by way of both the sixth and the seventh segmental arteries. The
position of the subclavian shows that it arises from the sixth segmental artery.
On the left side the subclavian slopes cranially as it leaves the aorta. It is evidently
being carried along by the shifting of the aorta, which just at this time has become
accelerated. It may be that this would have resulted in a breaking of the connection of the vertebral with the subclavian by way of the sixth segmental artery
and an enlargement of the latter by way of the seventh, thus restoring the usual
condition. Since the right subclavian will also after a time be drawn caudally,
a like readjustment of the vertebral on this side would have been possible.
The changing relation of the primitive subclavian to the aortic bifurcation is
significant in its development. Were the right vessel to come off below, it would
arise from the descending aorta in the adult. For a time after its appearance it
was always found to arise below the bifurcation. The distance of the origin from
the bifurcation is not great; but because of the irregularities in the position of the bifurcation at the time when fusion ceases, it is somewhat variable, and it is not
unlikely that an occasional subclavian may arise from a paired aorta even at the
time of its first appearance. The subclavians do not share in the descent of the
aorta but move cranially upon it as it descends. As a result, they are each soon
found to be coming off from the paired aorta of its side. In 4 embryos of the
branchial period, in which the pulmonary arch was complete, the subclavians of
3 were already cranial to the bifurcation. In all of 8 slightly older specimens the
subclavians came from the paired aorta?. Important in the shifting of the subclavians on the aorta? is the mooring of the vessel by its large vertebral branch which
is passing into the rudimentary vertebral foramen of the sixth cervical vertebra. Its comparatively large size is also a factor, for, as will be seen in the discussion of the development of the vertebral arteries, the segmental arteries lying
cranial to the subclavians, though having a similar relationship to the paired aorta?
at this time, are not able to move upward on these vessels and so are stretched and
finally interrupted.
In the post-branchial period the asymmetrical changes in the paired aorta?
involve their branches (the subclavians), and the history of the latter becomes
very different on the two sides. On the left the subclavian continues its movement
along the aorta; on the right this is rendered unnecessary by the interruption of
the right paired vessel close to the bifurcation. This also makes it possible for the
right fourth arch and the paired aorta caudal to it to become the proximal end of
the right subclavian, since there has already been an interruption of the right
paired aorta cranial to the fourth arch. A decrease of the right dorsal aorta
to a diameter equivalent to the subclavian has taken place before it was separated
from the aorta at its caudal end. Because the more cranial break occurs considerably earlier than the caudal, the sharp angle between the arch and aorta has
gone and the two vessels have formed a nearly straight channel before the aorta
has lost its lumen at its distal end. This distal interruption of the aorta is accompanied by abrupt changes in the arterial channel where the more primitive subclavian enters the aorta. Just before the wall of the involuting segment weakens,
and while it is exerting its maximum tension at this point, due to the pull from the
shifting aorta, the subclavian and aorta meet at a downwardly directed acute
angle. The degeneration of the wall of the disappearing aortic segment releases
its tension on the point of union of subclavian and dorsal aorta, thus permitting
this point to withdraw upward until the primitive subclavian passes obliquely
upward to go over into the aortic segment by a moderate curve (figs. 14, 15, 19).
By the time the costal and sternal rudiments have swung toward one another
and fused in the mid-line, the curves have disappeared and the fourth arch and
aortic territory of the subclavian are no longer distinguishable. In this manner a
channel of great tortuosity is reduced to a nearly straight segment at a time the
embryo is increasing only about 30 per cent in length. It must be accomplished
by a great slowing in the growth of its wall. Later, the forward and medial growth
of the ribs produces another marked curve just distal to the origin of the vertebral
artery, so that the part of the subclavian proximal to the rib forms a letter U (fig. 20). This is due to the fact that the part of the vessel distal to the vertebral
artery shares the movement of the ribs, while the proximal portion is held to its
original position by the vertebral artery and other structures. The entire subclavian now lies in nearly the same transverse plane, since its origin is about level
to the upper surface of the first rib.
The left subclavian stem in the early post-branchial period continues its earlier
ascent along the aorta and on up the aortic arch. It was seen, in following the
development of the arch, that this is truly a process of moving of the subclavian
relative to the wall of the parent trunk and not a mere shortening of the aortic
segment proximal to its origin. From the relation of the proximal end of the
vessel to the definitive aortic arch, just when the rapid descent is reaching its
completion in embryos 21 to 24 mm. in length, it is clear that this shifting is not
rapid enough to compensate entirely for the aortic descent. At this time the
subclavian is still separated at its origin from the left common carotid by a considerable segment of the arch, with which it makes an acute angle. In Jackson's
models of a 31-mm. and a 65-mm. human embryo, copies of which are manufactured
by Hammar, the subclavian is shown already lying close to the left common carotid
artery (figs. 16 and 22 to 25).
Basilar Artery
In the earliest work on the development of the basilar and vertebral arteries, His (1880) made the error of regarding the two vessels whose fusion produces the basilar artery as vertebral in nature and fixed this idea in the literature by designating them the cephalic vertebrals. Macalister (1886) concluded, apparently from a study of the chick, that these vessels are not homologous to the vertebrals but correspond to the system of vessels running along the surface of the nerve tube. De Vriese (1905) confirmed this in the rabbit. The so-called cephalic vertebrals of His are continuous with similar vessels along the anterior surface of the spinal cord. Sabin (1917) also has studied these vessels by the injection method. In chicks of about 27 somites she traced them from the subthalmic region to the caudal end of the cord and termed them the longitudinal neural arteries. She finds that they arise by the meeting of a prolongation of the internal carotid with a cranial extension of an anastomosis of segmental arteries under the midbrain. De Vriese claims that very early branches are found which extend from the proximal part of the internal carotid up to a point on the two neural arteries cranial to the part formed by anastomosis from the segmental arteries. It is possible that these also take part in the anastomosis, giving rise to the caudal part of the longitudinal neural arteries.
The character of the paired longitudinal neural arteries is apparently somewhat dissimilar in different species and perhaps also between the cerebral and the cord regions in the same type. Sabin describes them as originating in the pig and the chick in the form of a plexus on either side of the subthalamic and more definitely as a pair of single channels along the rest of their course. In her figures they appear not as a thickened band of plexus but as well-defined vessels. Sterzi (1904), using injections of the cord of the chick, described them as definite channels, but he did not find so great a differentiation in a number of mammals which he studied. His figure for the sheep shows them as rather large and approximately straight vessels in a thick plexus.
Evans (1909a) published a very full series of figures from injections of the cord of the pig, showing the paired condition and successive stages of fusion. Here we have to do as frequently with two or three vessels side by side as with a single enlarged vessel of the plexus. Taken together, these findings seem to imply specific differences in mammals in respect to their being supplied with continuous arteries running under the nerve-tube or -by longitudinal tracts made up of irregularly succeeding segments of vessels which are in some places double or even triple.
The caudal connections of the paired longitudinal neural arteries in man were
described by Zimmerman (1889) as the hypoglossal and first cervical segmental
arteries. Since Evans (1912), by tabulating the segmental arteries in man in
order of their appearance, finds the first occipital to appear first and the other
segmentals in the order of position, there can be little doubt, in the light of the
observations of De Vriese and Sabin on the development of the longitudinal
neural arteries in other forms, that all the cranial members of the segmental series,
as far back as the first cervical, contribute by anastomosis to the formation of the
longitudinal neural arteries.
De Vriese (1905), in her study of the rabbit embryo, has given the only description of the formation of the basilar artery from the paired longitudinal neurals. She states that the neurals first form strong transverse anastomoses and that the segment of the right or the left tract between two successive anastomoses disappears, so that the basilar is made up of successive segments taken irregularly from the right or left tracts. Sterzi (1904) earlier described and pictured this same process in the formation of the anterior spinal artery of the cord in the chick.
The preceding discussion of the literature shows that the longitudinal neural artery in mammals varies from a zone of enlarged vessels in a plexus to a single continuous channel. Its nature and relation to the development of the basilar in man have not been described.
Two models were made covering the time of formation of the basilar, and sections of earlier embryos, before the 22-somite stage, were studied. In a beautifully preserved 4-mm. embryo, in which the third arch had just become complete,
it was possible to distinguish longitudinal vessels in the region of the future basilar
artery. At this time there were already paired arteries continuous cranially
with the internal carotids and caudally traceable to the posterior third of the
hindbrain, where they were lost among capillaries. They were not much larger
in diameter than the vessels of the surrounding plexus and showed their probable
origin from it by numerous lateral branches and a slight tortuosity of course.
They, were most closely approximated in the medullary region, where they were
separated by a distance equal to about six times their diameter. In a still earlier
embryo, which had 22 somites, no vessels could be identified in the plexus under the brain. It is probable, however, that the injection method would have shown
at least parallel tracts of enlarged channels serving as their precursors, since Sabin
found this condition in a 19-somite pig.
The paired vessels were found to be connected for the first time by anastomoses
in the late fourth-arch stage. These are but slightly enlarged capillaries and are
most advanced somewhat caudal to the ear vesicles. By this time the cord has a
well-developed tract of enlarged capillaries on each side, which limit the corresponding plexus ventrally and mark off a ventrolateral non-vascular band along the cord.
This arrangement resembles the condition found by Evans on the upper surface
of the brain in the formation of the superior sagittal sinus.
Fig. 26, a and b. Successive stages in the formation of the basilar artery, partly by connecting up of irregularly alternating segments of the right and left longitudinal neural arteries and partly by a coalescence of the two. a, embryo No. 2841, 4 mm. in length; b, embryo No. 810, 5 mm. in length.
In a 4-mm. embryo (No. 2841) the formation of the basilar is well under way and the sixth arch is present. In figure 26 a, drawn from a model, two strong anastomoses can be seen about opposite the otic vesicle and some slender ones lying more caudally. Behind the strong anastomoses the right longitudinal artery has enlarged but the left is still uninterrupted. Cranially the left is not only the weaker but has lost its continuity. This enlargement of irregularly alternating segments of the two longitudinal arteries is what De Vriese and Sterzi found in other forms.
In the model of a somewhat later stage we find the basilar artery as a single vessel through most of its extent, though one large and several smaller islands are present (fig. 26 b). Between them it lies too far midway of the position of the previous longitudinal arteries for one to be confident that it is entirely made up from segments taken over from the paired vessels. The presence of small islands in the center of its caudal portion is also strong evidence that this portion was formed by coalescence of the two arteries. The dorsal aorta, which was found unquestionably to coalesce, had similar islands. The fact that the small islands are also in line with the large anterior island at the hypophysis, which undoubtedly has on either side unchanged segments of the original paired longitudinal vessels, also speaks for this method of formation.
The longitudinal neural arteries are described as approaching each other
before the formation of the basilar. The distance between their axes was compared
in a series of 7 embryos, from the time of their appearance to the establishment of
the basilar, to find whether this actually takes place, since a lateral movement of
vessels as immature as these seems highly improbable. The measurements were
made on models and Edinger projections and the values thus obtained divided by
the magnification. The interval between the axes of the vessels was found to
remain constant. It was therefore only their adjacent walls that approached,
due to the result of their increase in diameter just as found in the fusion of the
paired aortae.
In the early sixth-arch stage the earlier history of the basilar is still indicated
by the presence of occasional islands and an irregular, dorso-ventrally compressed
form. In a 12-mm. embryo, with subclavian well established, this condition had
passed. Tardiness in fusion was shown in a 14-mm. embryo by the persistence
of the paired condition for a considerable distance back of the isthmus. It is in
this manner that partly double adult basilars are formed.
The connection of first and second occipital segmental arteries and of more
cranial branches from the dorsal aorta with the longitudinal neurals is to be expected at an earlier period than is represented in our series. It was found that at
the time of formation of the basilar artery the hypoglossals, as well as the first
cervical segmentals, connected the dorsal aorta and longitudinal arteries, though
this is later than they have previously been observed. Since, in two instances,
the hypoglossal vessels were very slender and were lost in a capillary plexus for a
short distance, they were evidently just about to lose their identity. The first
cervical segmentals were of considerable size and clearly served to supply the blood
to the paired longitudinal arteries in their late stage and consequently are the
chief caudal connection of the basilar.
The continuity of the paired longitudinal neural vessels of the cranial region
with the longitudinal tracts of the plexus on the spinal cord was readily distinguishable before the time of formation of the basilar artery. It could not be seen from
the examination of cross-sections that a pair of definite vessels superseded the
tracts on the cord either before or after the establishment of the basilar.
Vertebral Artery
The formation of the vertebral artery is the most perfect example of the evolution of a longitudinal arterial channel from the segments of a series of transversely running arteries and the anastomoses between them. While the internal mamillaries and the inferior epigastrics of the trunk and the caudal portion of the longitudinal neural arteries of the brain arise in the same general manner, the close association of the anastomoses of the forming vertebral arteries with the vertebrae and other segmental structures gives them an unequaled regularity as to position and form. In the development of the vertebral, the capacity of the bloodcurrent to make a channel for itself by converting to its use segments of many different vessels is well shown and illustrates the hard and fast boundaries which the environment may place upon the course of a blood-stream. This mode of origin was already recognized for the vertebral by His in 1880. Froriep (1886), in connection with his description of the development of the vertebral column of the calf, showed the nature and relationships of the anastomoses. He figures them as passing from one cervical segmental artery to the next through an opening between the costal and the dorsal elements of the rudiment of the transverse process of the vertebra and lying medial to the spinal nerve. Hochstetter (1890) described a stage in the rabbit in which the anastomoses were large and swollen and the segmental arteries still complete. He noted that the proximal end of these vessels had been bent caudally by the shifting of the aorta in that direction.
The vertebral arteries come into being because the cervical segmentals are involved in the shifting of the neck structures on each other. The shifting of the cranial end of the nerve-tube relative to the digestive tube and other more ventral structures results in the cervical spinal ganglia and the spinal nerves after a while taking an oblique course ventrally and caudally. The segmental arteries also take on a similar direction (fig. 27). It is not certain whether the vessels would in time have become modified to allow the arterial current to pass upward more perpendicularly or whether the obliquity might have been permanently maintained. In any case a distinctly unfavorable condition develops for the segmental vessels at their proximal end due to the caudal shifting of the aorta. This affects only a short segment of the artery, since the more distal part is held in its intersegmental space by the condensed mesenchyme of the vertebral rudiments. As a result, the short proximal part takes on a much more oblique direction than the rest.A model of a 9-mm. embryo shows well this condition.
In the proximal region of their abrupt slope the second and fifth cervical segmental arteries form an angle of about 45 degrees with the long axis of the aorta. In the others of the series up to the seventh the slope is somewhat less. The segmentals are evidently exposed to unusual longitudinal tension in this region. The abrupt bending at either end of it, and more especially where it emerges from the aorta, must tend to greatly retard the current-flow. The vessels are here under conditions very unfavorable for further development or for even maintaining themselves. In this part of their course they are all of very slender caliber, or, at the end closest to the aorta, have become lost in the capillary plexus. It seems probable that their involution consists in a distribution of the endothelial cells of their wall among capillaries of the plexus that succeeds them and not in a cell degeneration. The part of each segmental distal to the bend is of much greater diameter, and, taken as a whole, the vessels have a characteristically conical form due to an increase in diameter as they pass dorsally. This is seen in Hochstetter's figure and is mentioned in Barniville's (1914) account of a human embryo. The sixth artery shows
much less slope, in both its proximal and distal parts, than the others. The seventh, of which the subclavian is a branch, is a robust vessel and comes off perpendicularly from the aorta. The reason for the dissimilarity between this vessel and the more cranial segmentals can be better understood after an examination of a slightly older stage.
In a second model of a 9-mm. embryo the vertebrals are midway in their
formation (fig. 28). Anastomoses are now established between all the cervical
segmental arteries except the first and second. A certain amount of variability in
the details of the formation of the vertebral is well illustrated by the equality in the
number of interrupted segmentals in this embryo and the other previously described,
taken with the dissimilarity shown by the two in the development of anastomoses.
Figs. 27 and 28. Stages in the formation of the vertebral artery. In figure 27 (embryo No. 721, 9 mm.), two segmental arteries arc interrupted but no anastomoses have yet formed between them. In figure 28 (embryo No. 143, 9 mm.), retrocostal anastomoses have formed between all but the first and second segmental arteries.
Also, it is not the same segmentals that are interrupted in the two embryos. The individuality in the story of the interruption of the successive arteries is clearly seen in their varying angles relative to the aorta and in the inequality of the intervals between their origins.
The anastomoses between the segmental arteries, with the exception of the first and second, are of a regular character, since they are situated among segmentally arranged vertebral nerves and arteries. They pass caudally from a segmental vessel, where it lies medial to and indented by a spinal nerve, and connect with a more distal part of the next succeeding artery. In this way a channel is developed from alternating anastomoses and segments from successive segmental arteries. The last contribute the larger amount to the vertebral artery. If the proximal ends of the segmentals are drawn up into the vertebral, as in the case of the interrupted divisions of the dorsal aorta, then a still larger fraction of its material must come from the segmentals. Corresponding to the oblique course of the latter, the vertebral artery presents a wavy or zigzag appearance when seen from the side and front. A spinal nerve lies in each laterally open notch. For some reason that was not determined, the anastomoses arch laterally for the short distance in which they are free of the spinal nerve.
We know from the course of the adult vertebral artery lateral to the anterior branch of the suboccipital nerve that the anastomoses between the first and second cervical segmental arteries must have this relation to the nerve, though more caudal anastomoses lie medially. The anastomosis at this point could not be followed from one artery to the other in any model, but it is evidently forming. It is not clear just why it passes lateral to the nerve. The environment of the first segmental differs from the surroundings of the others in that the spinal ganglion and nerve are small and separated by a wide gap from the next of their series. Other structural conditions connected with this and not so easily distinguishable evidently permit the arching of the anastomosis to reach its maximum at this segment.
The first cervical segmental arteries, as noted in the discussion of the basilar development, remain in connection with the paired longitudinal neural arteries after the more cranial segmentals have begun to separate from them. As they are still continuous with them at the time of formation of the vertebral artery, they serve to continue the vertebral of either side into the basilar. It was not determined whether or not a short caudal segment of the longitudinal neural arteries of the brain remains unfused on either side, but if it does it would furnish material for the distal end of the corresponding vertebral artery.
The vascular plexus on the lateral surface of the spinal cord and caudal end of the medulla undergo striking changes during the establishment of the vertebrals. Before anastomoses have developed we find the vessels turgid in the region of the first cervical ganglion. The segmental artery here is also much distended where it sends ramifications into the plexus of the medulla and cord. The distension of the plexus and segmentals soon extends farther caudally along the cord. This distension is perhaps due to the plexus and the distal part of the segmental arteries temporarily carrying the blood-stream which was formerly distributed to it under lower pressure from the arteries and which is soon to be taken over again by the vertebral artery. Though the walls of the capillaries have not as yet been shown to differ in strength from those of the segmentals, it is safe to assume from functional considerations that they are already weaker. It is to be expected, therefore, that, when the current of supply to the cervical cord is rather abruptly thrown directly into the plexus, the walls of its capillaries and of the distal ends of the segmentals as well should become stretched.
There is a caudal decrease in distention of the vessels and in the size of the anastomosis and a proximal tapering of the individual segmentals, which suggest that, while the vertebral is forming, the blood-stream to the more cranial part of the cervical cord is now coming by way of the basilar and internal carotid arteries. Sabin's observations, from injections in the chick, that the paired longitudinal arteries of the brain are formed by the meeting of a cranial branch from the internal carotids and a caudal vessel formed by the anastomosis of segmental arteries is reason for assuming that at the time of the establishment of the longitudinal neural arteries, at least, the carotid current passes backward under the fore part of the hindbrain. The comparative anatomical studies of De Vriese have shown that the supply of a large part of the brain by the vertebral current is an acquirement
of higher vertebrates. The internal carotids primitively reach the hindbrain. De Vriese believed that she could trace in sheep embryos a progressive change in the direction of tapering of the basilar artery of such a nature as to indicate that this vessel at first acted as a branch of the carotids but later as a part of the vertebral system.
It is difficult to establish differences in diameter of vessels at this early stage
because of then great distensibility, dependent upon conditions at death and later.
There was, however, some evidence that the diameter of the vertebral at the time
of formation was greater in its more cranial part. It seems probable, therefore,
from these various considerations, that before the formation of the vertebral artery
the hindbrain receives its chief supply from its cranial connections and that during
the formation of the vertebral the current may pass back into its territory. The
establishment of the vertebral must sooner or later put an end to this condition.
In the 14-mm. embryo the vertebral artery has acquired a nearly uniform
caliber (fig. 23). The proximal end of the subclavian, which was earlier the stem
of the seventh cervical segmental, has enlarged to the proper dimensions to carry
both the vertebral and the subclavian streams. The vertebral now comes off very
close to the aorta and it is distinctly larger than the subclavian. It is still nearly
as tortuous as at first. The maintenance of this condition in an artery for a considerable time is of great rarity, since all vessels tend to straighten out their angles
rapidly. It persists only because its surroundings force this course upon it. In a
21-mm. embryo (No. 448) the vessel is becoming straighter (fig. 25).
The vertebral artery is formed by the elaboration of material from so many
sources that it will be well to enumerate its components. Beginning at its origin,
there is a segment of the seventh cervical segmental artery distal to the origin of
the primitive subclavian artery. Next come short portions of segmentals 1 to 7
with the anastomoses between them. The first cervical segmental carries on the
vertebral channel from the lateral side of the first cervical vertebra to the caudal
ends of the ventral neural arteries of the brain as they lie under the medulla. As
has been said, it is not certain whether the ventral neural arteries themselves
contribute to it.
The chief cause of the interruption of the segmental arteries has been given as the tension of their proximal segments and their abrupt bending at each end due to shifting of surrounding structures. A further understanding of the changes ending in the vertebral formation may be gained from the seventh segmental, which did not succumb to these conditions. There are two circumstances which have probably contributed to its survival. The dorsal aorta, as was earlier shown, does not shift equally throughout its entire extent, in relation to its environment, but the movement begins at the cranial end. At the time of the formation of the vertebral artery the process has just reached the caudal end of the cervical region. It may be, then, that there has as yet been no shifting at the origin of the subclavian. If there has already been a slight movement, then we must infer that since the subclavian still comes off perpendicularly it must have moved along the aorta, just as we know it does in the succeeding phase of its development. It is not improbable that of the cervical segmentals it alone can do this, since it has a greater current than its companions, due to its supply of the limb-bud.
Because of its success in maintaining itself, it naturally falls heir to the distal territory of the more cranial segmentals by means of the anastomotic chain which connects them and which earlier seems to have supplied them with blood from the opposite direction. Its ultimate capture of the vertebral as a branch, as soon as time is given it to expand, is a natural sequel of its closer connection with the main arterial stream than is possessed by its rival, the internal carotid.
Summary
The evolution of the aortic-arch system is one of the most striking and complete instances of recapitulation in human development. The arches are not, however, all present at one time, as in many anamniotes, the first disappearing before the last arises. The arches develop as a result of the interposition of the pharynx with its pouches between heart and aorta in the early embryo. As soon as the heart moves away from the pharynx they disappear. The developmental period during which the arches are present may be termed the branchial-phase, and the remaining time, up to the attainment of the adult condition, the postbranchial phase K The interruption of the sixth arch was arbitrarily taken as marking the division between the two. The branchial phase occupies about 22 days and the post-branchial 28 years, yet the organization of the adult arterial system of the head, neck, and thorax is far along toward completion in the first 14 days of the post-branchial period.
The first arch has been found in mammals to develop from a preexisting angioblastic and capillary net. In man the arches are formed of sprouts converging
from the dorsal aorta above and the aortic sac below. These seem not to be preceded by a very complete net, though a sparse plexus does first grow out from a
bulging of the aorta and the sac. The sprouts, because of then rapid formation
and large size relative to the net, seem to develop as much from an outgrowth of
endothelium as by differentiation of the net already present. In the development
of the pulmonary arch the simple dorsally directed sprout from the sac does not
appear. Instead, there is an outgrowth of the same nature, directed caudally.
By fusion with a sprout from the dorsal aorta it is bisected into a proximal and a
distal part, the first forming the ventral end of the arch and the second the primitive pulmonary artery.
The arches develop in their order from before backward. The first undergoes
involution about the time the fourth is complete, and the second disappears before the pulmonary arch has become a continuous channel. Later, the third ceases
to send blood caudalward into the aorta. Thus the stream from heart to aorta is
shifted caudally in the branchial period. After the pulmonary arch is complete
there is a period of comparative stability of the arches, the fourth and pulmonary
delivering the blood caudally into the aorta, aided for a time by the third. The
length of the third and fourth arches is almost constant throughout their existence,
because their form is dependent on the caudal portion of the pharynx, which increases in size very little after its establishment and early shows regressive changes.
Fifth Arch
Loops, or so-called "island-formations," appear sometimes in the angioblastic and capillary net which precedes the upper end of all arches but the first. Occasionally they are found at the lower end. Those found at the upper end of the fourth and pulmonary arches have been incorrectly classed with other arterial channels which bear a real resemblance to a fifth arch. If one omits the islandformations, 6 cases of so-called fifth arches have been described in man. In 5 of these it arose from the lower end of the fourth arch or the subjacent sac and passed to the upper end of the pulmonary arch. Lewis and Kingsbury point out that even the occasional existence of a fifth arch can not be regarded as established, since the identity of this structure depends on its lying in a fifth visceral arch, and this has never been proved. Yet there is some evidence of the occasional occurrence of the latter in a more or less complete form. Projecting from the aorta and sac are frequently found sprouts corresponding to the upper and lower ends of the fifth arches. Taken with the complete vessels, these were found in 50 per cent of the embryos representing the time of establishment of the pulmonary arch and a little later. It can not be said how many of these are developing so-called fifth arches, how many are stages of regression, and how many are incompletely developed so-called arches which never will progress farther.
The enlargement where the arches come off from the arterial trunk, which we have termed the aortic sac, is already present when the second arch is forming.
It is best developed when the three pairs of arches are coming off from it and remains for a time after the pulmonary arches and trunk are cut off. A similar
structure is found among anamniote embryos, and a sac of similar form and position is observed in some adults of the same group. Perhaps the embryonic like the
adult sac either serves to distribute the diastolic pressure or is a mechanical adaptation to the forces resulting from the rapid deflection of the current from the arterial
trunk into the arches. Dr. Streeter suggests, as a purely developmental explanation for its presence, that it may be due to an excessive proliferation of endothelium
which is to be used up later in differentiation of the arteries.
Most writers describe paired ventral aortae in the human embryo. There are at different times a few temporary channels leading from the sac which, by their approximately cranio-caudal course, resemble fragments of ventral aortae. Such are the longitudinal segments that appear in the late history of the first and second arches and the paired sprouts which give rise to the proximal parts of the pulmonary arches and the primitive pulmonary arteries. These vessels are truly indicative of a general structural plan which in some lower vertebrates is elaborated to a degree that permits the development of paired ventral aorta?. There is no phase of human development, however, in which such vessels exist.
Involution of First Two Aortic Arches
After the disappearance of the first and second arches, their corresponding visceral arches are each occupied by two successive channels; these may be termed, respectively, the earlier and later mandibular and hyoid arteries. The earlier the near the axis of the arch ; the later run close to its caudal border. These arteries all break up in a plexus in the subpharyngeal region. Their current is from the dorsal aorta, and they supply the visceral arches. Only the later hyoid artery could be found in the post-branchial period, when it constituted the stem of the stapedial artery. There was no evidence of cell degeneration during the involution of the arches. Small endothelial saccular enlargements or lacunae were found in the subpharyngeal region after the arches had disappeared, but these were always parts of vessels. They may be due to proliferation of endothelium later to be used in the rapid differentiation of vessels.
The precursors of the external carotid arteries are seen, soon after the first and second arches have gone, as a pair of irregular and inconstant sprouts from the aortic sac. They sometimes send branches into the bases of the mandibular and hyoid arches. They first he near the mid-line, but gradually they either move lateralward or are replaced by more lateral vessels. In the earlier part of the postbranchial period, when the identity of the third arch is becoming lost, these arteries are found coming off from the middle of the third arch. Lingual, thyroid, and other branches are distinguishable at this time.
Principal Changes during Post-branchial Phase
The early post-branchial phase is the time when rapid disintegration of the arch system takes place. Since the identity of its parts is largely topographic, their walls differing little in structure, one can not expect to trace the parts, as hard and fast units, into the later vessels. It is of interest, however, to learn in what manner the earlier vessels give up their identity in the mature arteries which evolve from them, since this subject in any part of the vascular system seems to have received little attention.
The rapid breaking up of the arch system is effected principally by its interruption in four places. The loss of the segment between the dorsal aorta on either side, between the third and fourth arches, helps especially in the formation of the carotids. The cutting off of the right paired aorta at its caudal end makes possible the completion of the right subclavian artery. The degeneration of a part of the right pulmonary arch permits the development in the fetus of the main pulmonary channel, the two parts of which are termed the definitive pulmonary artery and the ductus arteriosus.
In each instance one immediate cause of the interruption seems to be a reduction of current-flow. In the two aortic segments between the third and fourth arch it is due to a stagnation at this point, the streams in the aortic segments cranial and caudal to it flowing in opposite directions. This in turn may be ascribed to the rapid increase in the mass of the head region and the consequent enlargement of the territory supplied by the cranial end of the aortae. The right pulmonary
artery receives less current than the left, because it is a less direct route to the aorta, due to the pulmonary trunk ending to the left of the mid-sagittal plane. The stream to the right paired aorta caudal to the fourth arch is reduced, because the sixth arch on this side has become interrupted and the aortic trunk is at this time directing its current more into the left than into the right fourth arch. The short interval of the aorta between the subclavian and the bifurcation suffers a still greater reduction of current, as it does not carry the stream to the subclavian.
The downward pull exerted by the descending heart upon the derivatives of the arch system also probably contributes to these interruptions. That the effect of tension is less important than current-strength is shown by a comparison of the right pulmonary arch and of the right end of the right aorta with the corresponding regions on the left side, since the latter were likewise exposed to the tension yet did not succumb. The excessive tension at least hastens the degeneration, therefore, after it is once begun. The regions affected first contract and then are pulled out into long filaments. These in turn are broken. With the exception of the pulmonary arc whlch leaves behind a long cord ol degenerating cells, the recoil of the broken filaments brings back most of the substance of the involuting region to a position close to the adjacent vessels, where it is worked over into their walls.
The development of the definitive aortic arch is complex, since its material comes from many sources. In a 14-mm. embryo the last trace of the division of the dorsal aorta between the third and fourth arches is just about to disappear, but the ends of the arches are still defined. Since the aortic arch is just taking form, one can learn the sources of its respective regions, and it is seen that the aortic trunk, left half of aortic sac, left fourth arch, left dorsal aorta between the fourth and pulmonary arches, and the part of the aorta lying next most caudally lose their identity in it. The right half of the sac elongates to become the innominate artery. The right and left third arches, as far up as the external carotids, develop into the common carotid arteries.
The history of regions of the forming definitive aortic arch may be inferred by a study of measurements of the divisions of the arch system in the late branchial period and the parts of the arch apparently arising from them. The segment between the innominate and left common carotid, originally equivalent to the left half of the aortic sac, keeps pace with the growth of the body-length until the heart and arch, make their rapid d escent into the thorax at the stage of 14 to 18 mm. At this time the in nominate and left common carotid approach. This is almost certainly due to the origin of the innominate moving distally on the arch. The proximal part of the arch, as far as the innominate, has been elongating steadily, but its sudden extension, as the arch rapidly descends, is probably due to the origin of the innominate moving distally through the more distal region of the definitive arch as far as the ductus arteriosus. As a result, the fourth-arch region, which was at the summit of the forming definitive arch, sinks to the descending limb, and the region of the left common carotid, now shared by the innominate, comes to constitute the entire summit. The region of the arch derived from the fourth arch increases rapidly in diameter to reach in cross-section an approximate equality with the more proximal and distal parts derived from vessels which are already capacious at the beginning of the branchial period. The. arch as a whole increases little in length, but considerably in circumference, up to the 24-mm. stage.
The definitive arch in its early history lies in almost the mid-sagittal plane, because at its distal end the aorta has not taken a paravertebral position and at its proximal end the heart has not yet come to he obliquely in the thorax. The arch has a large radius before the closing of the superior thoracic aperture by the meeting in the front of sternal bands and ribs. Then, due to the swinging caudally of the apex of the heart to accommodate itself to the decreased space of the thoracic cavity, it is bent rather sharply at its summit.
The innominate and common carotids swing into a nearly longitudinal position during the rapid descent. They still slope somewhat ventrolaterally in the 24-mm. embryo as they pass upward, because of the large size of the head relative to the
body. The innominate lengthens to about the same degree as it decreases in circumference; relative to the increasing body-length it is much longer proportionally in the 24-mm. embryo than it is in the adult. The common carotid arteries extend
rapidly coincident with the rapid descent of the heart. It is not clear how much of this is due to the elongation of the region from the proximal half of the third arch and how much to the arch being pulled caudalward, thus forcing the external
carotid to shift cranially along its wall and the wall of the dorsal aorta cranial to it.
Changes in Topography of Aortic-Arch System
The displacements of the parts of the arch system and of the aorta, due to the unequal growth of different organs, are chiefly longitudinal and transverse. The paired primitive aortae grow toward each other in a part of their course and are carried apart in other regions. The approach is in the thoracic region and is not a movement of the vessels as a whole toward each other, but merely an approximation of their contiguous walls due to the increase in diameter of the vessels. It is permitted by the withdrawal of the nerve-tube and notochord from the digestive tract.
The fusion of the aortae takes place by the enlargement of capillaries lying between the vessels to form transverse anastomoses. These then fuse so that a unit vessel results with a cross-section like the figure 8. This in turn is remolded to the ordinary arterial form. The fusion begins somewhat back of the cervical region and progresses both cranially and caudally. It comes to a stop about 4.5 body segments caudal to the pharyngeal territory, where the pulmonary arch is forming. Due to the growth displacement of the cranial end of the nerve-tube relative to the pharynx, the most cranial point of fusion soon after the process has begun is opposite the second cervical ganglion; while later, when fusion is complete, though it has moved forward relative to the pharynx, it is opposite the seventh ganglion.
Fusion does not progress farther cranially because the developing cervical vertebrae and the digestive tube, pressing upon the aortae from above and below, tend to crowd the latter apart. If we take the paired aortae in connection with thencranial extensions, the longitudinal neural arteries, we find that during growth these two vessels, at first nearly parallel, are carried widely apart in three places and to only a slight extent in two intermediate regions. The two vessels together produce
a trilobed figure. The most caudal separation, which is also the least in extent, is due to the pressure of the cervical vertebrae and digestive tube, as already described. The next is over the anterior part of the pharynx and is the result of the
rapid widening of this region. The third is on the forebrain and is produced by the rapid lateral expansion of the latter. At the caudal end of the pharynx is a region in which the arteries are in close approximation. The pharynx here is in a condition of regression and increases in width very slowly. It may be that the pressure of the vagus nerve on the lateral side of the vessels here, as it passes downward across them, has some effect in preventing their being carried apart, since they sometimes show its impress. Just cranial to the pharynx another approximation is due to a less rapid increase in width here than is shown by the forebrain and pharynx lying, respectively, cranial and caudal to it.
The paired aortae and a part of the unpaired vessel shift backward relative to their immediate environment, the pharynx and digestive tube. The shifting first occurs at the cranial end of the aortae, since here they first fall behind the surrounding structures in longitudinal growth. The withdrawal then takes place progressively in more and more caudal parts of the paired aortae and then involves the unpaired aorta to an increasing extent. In the earlier part of the branchial period, when fusion of the aortae has just been completed, the withdrawal has not progressed to the fusion point, but is shown only by the bending backward of the dorsal ends of the more caudal arches soon after each appears. The next indication is a sharp forward bend of the proximal end of the cervical segmental arteries as far back as the sixth. The aortic bifurcation remains at rest for a while, but the region of withdrawal has extended back to it at about the end of the branchial period.
Beginning in embryos of 14 mm., there is a rapid caudal shifting of the point of bifurcation, which ends at about the 17-mm. stage. The caudal movement continues and is not complete in the 24-mm. embryo, in which the superior thoracic aperture is closed and the heart is in the thorax.
The fusion of the paired aortae also progresses caudally as well as cranially. The caudal paired vessels are always very short, for while they are elongating distally they are fusing proximally. The paired condition, unless perhaps in the form of very slender terminals, does not remain at this end of the body. In 4 to 6 mm. embryos only very short double vessels are present and later no definite aortae could be recognized. The distance which the territory of the aorta derived from the bifurcation shifts can not be told precisely. There is a type of anomalous right subclavian artery, however, which evidently taps the aortic system by retaining the caudal end of the right paired aorta, since in the adult it comes off as the distal branch of the aortic arch. Inasmuch as it has been found coming off as low as the fifth thoracic vertebra, there can be little doubt that the region of the aorta derived from the bifurcation also moves down this far. In fact, it is not unlikely that the subclavian has moved up the aorta somewhat from the region corresponding to the bifurcation, since the left subclavian regularly does so. It is known that the caudal end of the aorta withdraws cranially. There must, therefore, be a point intermediate between the ends which remains more or less fixed in relation to its immediate environment, and this point must be in the proximity of the thoracicolumbar boundary.
The interruption of the right paired aorta at its caudal end is quickly followed by a shifting to the left of that part of the definitive aortic channel just back of the point of the former bifurcation, as the angle between it and the right paired aorta is straightened. This is a step in the movement of the thoracic aorta to its final position in the left side of the vertebral column. It is probable that a pressure
analogous to that which crowded apart the paired aortse acts later to push the definitive unpaired aorta to the left.
The shifting of the aorta is paralleled by a caudal displacement of the arches, their ventral connections, and the heart itself. The arches not only shift at their upper ends as far caudally as possible, but the entire fourth arch is curved around the caudal pharyngeal complex at the end of the branchial period, as though this mass were resisting its caudal progress. In a similar way the loop formed by the vagus and recurrens nerves presses against and molds the pulmonary arches. The aortic sac also shifts correspondingly. In the post-branchial period the interruption of the various parts permits a rapid descent of heart, arch, and other vessels. The fourth arch moves, relative to the pharynx, about 4-I2 body segments. Since the nerve-tube grows forward relative to the pharynx, the arch moves on the nervetube about 13 body segments. During the period of rapid descent (embryos 14 to 18 mm.), the arch moves at a rate of about one-fourth of a segment a day. This displacement is the continuation, in another guise, of the shifting of the blood-stream from heart to aorta, which, in the branchial period, was effected by the loss of the cranial arches and the development of new caudal ones.
The heart changes its relation to the sac during growth. At first the arterial trunk approaches the sac from a cranial direction, indicating that the apex of the heart is pointing forward. At about the end of the branchial period we find the apex of the heart pointing in the opposite direction, so that the arterial trunk reaches it from its caudal side. The long axis of the heart is at right angles with the perpendicular axis of the body at about the time (near the end of the branchial period) when the proximal ends of the fourth and pulmonary arches are well apart. Therefore, it may be that the heart crowds against these arches at this time and pushes them apart.
The downward movement of the heart, sac, and arches, like the retreat of the aorta, is due to the failure of the heart and certain territories caudal to it to keep pace with the longitudinal growth of other adjacent parts of the body. The descent of the heart causes a movement of other structures to fill in the space vacated by it, such as the pharyngeal derivatives and probably mesenchyme. The arteries, however, probably have a more active role, and they themselves, while pulled by the heart, actually aid the caudal movement of some of the other structures by pressure against them. The moving in of structures to take the place of the heart has been aptly termed by Kingsbury a "growth eddy." The body-wall takes an active part in the eddy, as indicated by the change in position of the rib rudiments and sternal bands. The ribs, before the rapid descent, point upward and outward at an angle of 90 degrees with the sagittal plane. They sink caudally at their distal ends, and by the development of a curve and an increase in length they come to form, with the aid of the sternal bands, an arch which completes the thoracic inclosure on its ventral side. This process follows quickly upon the descent of the heart into the thorax.
The heart and large vessels change their position just in time to accommodate themselves to the restricted quarters resulting from the closure of the superior thoracic aperture. The kinking of the aortic arch, which occurs at this time, results in a dorsoventral diameter commensurate with the size of the aperture. The left subclavian and the innominate are now near the summit of the arch, so that the arches of the branch are well placed to find exit from the thorax.
The arterial changes that have been recounted include many illustrations of apparent effects of the longitudinal pull of the heart and. dorsajaorta on the arteries with which they are connected, usually acting~aTone~but sometimes associated with other causes. Among these may be mentioned the involution, the stretching threads and the breaking of segments of the arch system, the caudal movement of vessels, swinging of vessels into an approximately longitudinal direction, especially rapid growth of arteries during the descent of the definitive arch, and the movement of the subclavian and innominate along the vessels of origin. Experimental evidence will be necessary to establish definitely the action of longitudinal t ension in most of these cases, but even in the absence of light from this quarter the developmental picture offers strong indications in its favor.
Pulmonary Artery
The pulmonary artery takes origin when the sprout from the dorsal aorta caudal to the caudal pharyngeal complex establishes a connection with the caudally extending sprout from the aortic sac, thus dividing the latter into two parts - a proximal portion, which becomes part of the pulmonary arch, and a distal portion, the primitive pulmonary artery. The sprout from the sac is preceded by a welldeveloped plexus, which itself has sprung from the sac and seems more to be the result of the elaboration of the endothelium of the plexus than were the other aortic-arch sprouts.
When the right pulmonary arch becomes interrupted dorsal to the origin of
the right pulmonary artery, the angle between the artery and the proximal segment
of the arch straightens out, so that the arch remnants become a part of the artery.
Similarly, the angle between the left arch and pulmonary trunk becomes rectilinear,
so that these two elements form a large trunk, slightly curved, which extends from
the pulmonary side of the heart to the distal end of the aortic arch. In the straightening of this angle, the origin of the right pulmonary is carried ventrally, and near its
origin the vessel becomes somewhat curved about the proximal end of the aortic arch.
The segment of the main pulmonary vessel between the origin of the right and left
pulmonary arteries shifts away from the heart and toward the aorta. It is not
certain to what degree this is due to inequality in the growth of the segments
proximal and distal to the vessels and to what degree it is a matter of movement
of the origins of the two vessels in the wall of the main stem. Several things,
however, point to its being due chiefly to the former cause. During the period of
rapid descent, the points of origin of the two pulmonary arteries rapidly approach
each other. This must be due to the movement of one or both through the substance of the main vessel. They meet before the 40-mm. stage. The manner in which they come together does not favor the view that they have become wrapped
about the pulmonary stem by means of its rotation and, by fusion with it, gradually approach each other at their points of origin. After coming together the arteries are designated by their adult terminology - right and left branches of the pulmonary artery. The main stem proximal to them is the pulmonary artery, and the channel from the origin of the left pulmonary to the dorsal aorta is the ductus arteriosus.
Subclavian Artery
In their development the subclavian and vertebral arteries show to an unusual degree the capacity of the blood-stream to take over and mold into a unit a number of previous channels. Th e subclavian artery can first be recognized at the time the forelimb-bud is but a slight elevation, after the completion of the fourth arch and before the pulmonary arch is complete . It is at this time a slender channel lying in the sixth cervical intersegmental space and coming off from a sac-like projection of the aorta which later develops into the stem of a segmental artery. There are similar vessels in adjacent intersegmental spaces which usually end in a plexus before reaching the limb-bud. It seems probable, as far as can be determined from the study of sections, that a second artery may occasionally extend into the limbbud, but this was not possible to ascertain with certainty in the absence of injection of in toto preparations. Since the vertebral artery arises from the subclavian, it usually enters the transverse process of the sixth cervical vertebra. The situation of the origin of the subclavian and vertebral a segment more cranial than usual is of much more frequent occurrence in the embryo than in the adult. There is evidence of a regulation, in a sense, back to the usual type.
The subclavian first passes to the limb-bud on the dorsal side of the brachial plexus, but later it is inclosed by an outgrowth of neurons over its dorsal surface. In a 14-mm. embryo one can distinguish radial, ulnar, interosseous, and some digital arteries, as well as different nerves of the limb. The subclavian soon incorporates, as a part of itself, the stem of the segmental from which it arose. At this time its segmental part comes off the unpaired aorta. As the aorta shifts caudally, the subclavian is moored by the vertebrals, and their other branches move each onto the corresponding paired aorta. At the time of rapid descent the right paired aorta is interrupted just where it goes over into the unpaired aorta and the subclavian comes off just above the bifurcation. Before the interruption, as was earlier explained, the right fourth arch and the paired aorta distal to it had already been reduced to a mere channel of supply to the subclavian. The interruption of the right aorta distal to the subclavian is the final step in giving these two segments over as the proximal end of the right subclavian.
The left subclavian continues its movement up the aorta and arch until, in the 17-mm. embryo, in which the aortic arch is complete, it is but a little way from the summit.
Basilar Artery
Paired longitudinal arteries develop along the lower surface of the brain and are continuous at their anterior ends with the aortse in the human embryo just after the establishment of the fourth arch. They were still incomplete in a 4-mm. embryo in which the fourth arch had just formed. Only the first cervical and second occipital (hypoglossal) arteries have been seen connected with the caudal end of these paired longitudinal arteries, but this part probably arises as anastomoses between all segmental arteries cranial to the second cervical, followed by a loss of the connection of these vessels with the aorta. The paired neural arteries were traced caudally into paired longitudinal arterial tracts of the cord.
The contiguous walls of the paired longitudinal neural arteries approach, as in the case of the primitive aortse, merely by enlargement and not by actual movement of the vessels toward each other. Cross anastomoses develop from enlarged capillaries ; and in the more cranial part of the region destined to be occupied by the future basilar artery, successive segments, taken irregularly from one or the other neural artery with cross anastomoses, are remolded into the basilar. Near the caudal end there is apparently a fusion of the two neural arteries to form the basilar. By the time the pulmonary arch is established, the formation of the basilar is well under way.
Vertebral Artery
There would never be a vertebral artery did not the aorta shift caudally. Its movement is responsible for the proximal ends of the segmentals, back to the seventh cervical, becoming stretched, decreased in diameter, and bent obliquely on the aorta. The more distal part of the segmentals also takes on a slope which is less abrupt and due to the shifting of the nerve-tube on the digestive tract and adjacent structures. It may be that this, too, is unfavorable to their maintenance. The seventh segmental, being larger than the more cranial vessels, due to its subclavian branch and because it lies in a region where there is as yet little caudal movement of the aorta, does not become oblique or constricted in diameter and does not degenerate.
Anastomoses develop between the successive cervical segmental arteries in the 9-mm. embryo. These pass caudally from one vessel and connect with the more distal part of the next succeeding member of the series. A channel is thus developed from alternating anastomoses and segments of segmental arteries. Of the two, the arteries contribute the most. The resulting vessel is tortuous in both sagittal and frontal planes. Due to the confined space in which the artery runs, it is not entirely straightened out even in a 52-mm. fetus. Each anastomosis arches laterally where it lies free of the corresponding nerve. That between the first and second segmental arteries extends more laterally than the others, so that it passes external to the suboccipital nerve. The part of the first cervical segmental artery distal to the anastomoses, up to its ending in the longitudinal neural artery, also becomes part of the vertebral. It was not decided whether the caudal end of the longitudinal neural also supplies material for the distal end of the vertebral or whether they all go into the basilar.
There are a number of considerations pointing to a cranial source of the blood passing through the entire basilar and the cranial part of the cervical cord at the time the vertebral is forming. It is probable, though less certain, that at an earlier period the longitudinal neural arteries also were supplied nearly to their caudal ends by the current passing forward into the neurals from the cranial end of the aortas. Where the vertebral is forming, the plexus on the cranial part of the cervical cord is turgid and the segmental arteries have a characteristically swollen appearance at their distal ends. They taper proximally to enter the aorta by a slender channel or to terminate in a plexus at this end. The current formerly borne by the cervical segmentals is apparently rather abruptly thrown into the plexus and the distal ends of the segmentals, due to the interruption of their proximal ends. As it is of higher pressure than usually carried by them, their walls are stretched. They are relieved by the establishment of the vertebral artery.
The subclavian artery, because it does not succunfb to the unfavorable conditions which cause the disappearance of the more cranial segmental arteries, and because it has a more direct connection with the main arterial stream than the cranial ends of the longitudinal neurals, falls heir to the anastomotic chain, thus making the vertebral its branch. The course of development of the human vertebral is in accord with the claim of De Vriese that in vertebrate phylogeny the brain is first supplied by the internal carotids and that only later does its caudal part come to be supplied by the vertebral.
Description of Plates
Plate 1
Figs. 29 and 30. Ventral and lateral views of the cranial portion of the arterial system of a 22-somite embryo. The first arch is at its maximum development and the dorsal and ventral outgrowths, which are to aid in the formation of the second, are just appearing. Embryo No. 2053, length 3 mm.
Figs. 31 and 32. Ventral and lateral views of an embryo in which the first arch has gone, the second arch is much reduced in diameter, and the third arch well developed. Dorsal and ventral outgrowths for the fourth and probably for the pulmonary arch are present. Embryo No. 836, length 4 mm.
Plate 2
Figs. 33 and 34. Ventral and lateral views of a 5-mm. embryo (No. 1380). The third and fourth arches are in a condition of maximum development and dorsal and ventral sprouts of the pulmonary arch have nearly met. The primitive pulmonary arteries are already of considerable length.
Figs. 35 and 36. Ventral and lateral views of an 11-mm. embryo (No. 1121). The pulmonary arches are complete and the right is already regressing. The third arch is now bent cranially at its dorsal end and its stream is about to become deflected in that direction.
Plate 3
Figs. 37 to 39. A 14-mm. embryo (No. 940) in which the last indications of the aortic-arch system are just disappearing and a very primitive condition of the larger arteries derived from them is already recognizable. Figure 37, lateral view; figure 38 ventrolateral view; figure 39, ventral view.
Fig. 40. Ventral view of an 18-mm. embryo (No. 1390). The arterial evolution has preceded so far that the adult vessels are easily recognizable.
Bibliography
v. Baer, K. E., 1828. Ueber Entwicklungsgeachichte der Thiere. Kbnigsburg.
Barniville, H. L., 1914. The morphology and histology of a human emljiyo of 8.5 mm. J. Anat. and Phys., vol. 49, 1-61.
van Bfmmelen, J. F., 1886. Die Visceraltasehen und Aortenbogen bei Reptilian und Vogeln. Zool. Anz., vol. 9.
Bremer JL. On the origin of the pulmonary arteries in mammals. (1902) Amer. J Anat. 1(2): 135-
Bremer JL. II. On the origin of the pulmonary arteries in mammals. (1909) Amer. J Anat.
Bremer JL. The development of the aorta and aortic arches in rabbits. (1912) Amer. J Anat. 13(2): 3-128.
Broman, I., 1898. Die Entwicklungsgeschichte der Gehorknochelchen beim Menschen. Anat. Hefte, vol. 11, 509-668.
Buell, C. E., Jr., 1922. Origin of the pulmonary vessels in the chick. Contributions to Embryology, vol. 14, No. 68.
Bujard, Ero., 191.5. Description d'un embryon humain (Eternod-Delaf) de 20 somites, avec flexion dorsale. Internal Monatschr. Anat. u. Phys., vol. 31, 238-268.
De Vriese, B., 1905. Sur la signification morphologique des arteres cerebrates. Arch, de Biol., vol. 21. 357-457.
Evans HM. On an instance of two subclavian arteries of the early arm bud of man and its fundamental significance. (1908) Anat. Rec.
Evans, H. M., 1908. On an instance of two subclavian arteries in the early arm-bud of man and its fundamental significance. Anat. Record, vol. 2,411-424.
Evans HM. On the earliest blood-vessels in the anterior limb-buds of birds and their relation to the primary subclavian artery. (1909) Amer. J Anat. 9: 281-319.
Evans HM. On the development of the aortae, cardinal and umbilical veins, and the other blood vessels of vertebrate embryos from capillaries. (1909) Anat. Rec. 3: 498-518.
Evans HM. The development of the vascular system. In Keibel F. and Mall FP. Manual of Human Embryology II. (1912) J. B. Lippincott Company, Philadelphia. pp570-708.
Fedorow, V., 1910. Ueber die Entwickelung der Lungenvenc. Anat. Hefte, vol. 40, 529-603.
Froriep, A., 1886. Zur Entwicklungsgeschichte der Wirbelsiiule, insbesondere des Atlas, Epistropheus und der Occipitalregion. II. Beobachtvmg an Saugethierembryonen. Arch. f. Anat. u. Phys., Anat. Abth., 69-149.
Gage SP. A three weeks' human embryo, with especial reference to the brain and nephric system. (1905) Amer. J Anat. 4: 409-443.
Goppert, E., 1909. Ueber die Entwicklung von Varietaten im Arteriensystem. Untersuehungen an der Vordergliedmasse der weissen Maus. Morphol. Jahrb., vol. 40, 268-410.
Goette, Alex, 1875. Die Entwicklungsgeschichte der Unke (Bombinator igneus) als Grundlage einer vergleicheri^ien Morphologie der Wirbelthiere. Leipzig.
Greil, A., 1903. Ueber die Entwickelung des Truncus arteriosus der Anamnier. Erganzh., Anat. Anz., vol. 23, 91-105.
His, W., 1880. Anatomie menschlicher Embryonen. Leipzig.
Hochstetter, F., 1890. Ueber die Entwickelung der A. vertebralis beim Kaninchen nebst Bemerkungen fiber die Entstehung der Ansa Vieussenii. Morphol. Jahrb., vol. 16, 572-586.
Huntington GS. The morphology of the pulmonary artery in mammalia. (1919) Anat. Rec. 17: 165-190.
Jordan HE. Description of a 5 mm human embryo. (1909) Anat. Rec. 3:
Keibel, F., and C. Elze, 1908. Normentafeln zur Entwicklungsgeschichte der Wirbeltiere. Heft 8, Normentafeln zur Entwicklungsgeschichte des Menschen. Jena.
Kingsbury BF. The development of the human pharynx. (1915) Amer. J Anat. 18(3): 329-397.
Kingsbury BF. On the so-called ultimobranchial body of the mammalian embryo. (1915) Anat. Anz. 47: 609-627.
Khanichfeld, H., 1914. Die iunktionelle Anpassungen der embryonalen Aortenbogen. Arch. f. Entw. der Organ., vol. 40, 668-671.
Krause, W., 1868. Handbuch der Gefasslehre des Menschen, vol. 3, erste Abtheilung-Gefasslehre.
Lehman, H., 1905. On the embryonic history of the aortic arches in mammals. Anat. Anz., vol. 26, 406-424.
1906. The fifth and sixth aortic arches and the related pharyngeal pouches in the rabbit and pig. Anat. Anz., vol. 28, 506-513.
Macalister, A., 1886. The morphology of the arterial system in man. Am. J. Phys., vol. 20, 193-200.
Mall FP. On the development of the human heart. (1912) Amer. J Anat. 13: 249-298.
Rabl, H, 1907. Die erste Anlage der Arterien der vorderen Extremitaten bei den Vogeln. Arch. f. rnikr. Anat., vol. 69, 341-388.
Rathke, H., 1843. Ueber die Entwickelung der Arterien, welche bei den Saugethieren von den Bogen der Aorta ausgehen. Arch. f. Anat., 270-302.
Rose, G, 1890. Beitrage zur vergleichenden Anatomie des Herzens der Wirbelthiere. Morphol. Jahrb., vol. 16,27-96.
Sabin FR. Origin and development of the primitive vessels of the chick and of the pig. (1917) Contrib. Embryol., Carnegie Inst. Wash. 6: 61–124.
Sabin, F. R., 1917. Origin and development of the primitive vessels of the chick and of the pig. Contributions to Embryology, vol. 6, Carnegie Inst. Wash. Pub. 226, 61-124.
Shaner, R. F., 1921. The development of the pharynx and aortic arches of the turtle, with a note on the fifth and pulmonary arches of mammals. Am. J. Anat., vol. 29, 407-420.
Stahel, H, 1886. Ueber Arterienspindeln und iiber die Beziehung der Wanddicke der Arterien zum Blutdruck. Arch. Anat. u. Phys., Anat. Abth.. 45-63.
Sterzi, G., 1904. Die Blutgefasse des Riickenmarks. Untersuehungen iiber ihre vergleichende Anatomie und Entwickelungsgeschichte. Anat. Hefte, vol. 24, 1-364.
Tandler, J., 1902. Zur Entwicklungsgeschichte der Kopfarterien bei den Mammalia. Morphol. Jahrb., vol. 30, 275-373.
1909. Ueber die Entwickelung des V. Aortenbogens und der V. Schlundtasche beim Menschen. Anat. Hefte, vol. 38, 393-423.
{{Ref-Thyng1914}
Thyng, F. W., 1914. The anatomy of a 17.8 mm. human embryo. Am. J. Anat., vol. 17, 31-112.
Woollard, H. H, 1922. The development of the principal arterial stems in the forelimb of the pig. Contributions to Embryology (this volume).
Zimmerman, W., 1889. Ueber einen zwischen Aorten- und Pulmonalbogen gelegnen Kiemenarterienbogeu beim Kaninchen. Anat. Anz., vol. 4, p. 720.
Historic Disclaimer - information about historic embryology pages |
---|
Pages where the terms "Historic" (textbooks, papers, people, recommendations) appear on this site, and sections within pages where this disclaimer appears, indicate that the content and scientific understanding are specific to the time of publication. This means that while some scientific descriptions are still accurate, the terminology and interpretation of the developmental mechanisms reflect the understanding at the time of original publication and those of the preceding periods, these terms, interpretations and recommendations may not reflect our current scientific understanding. (More? Embryology History | Historic Embryology Papers) |
Glossary Links
- Glossary: A | B | C | D | E | F | G | H | I | J | K | L | M | N | O | P | Q | R | S | T | U | V | W | X | Y | Z | Numbers | Symbols | Term Link
Cite this page: Hill, M.A. (2024, April 27) Embryology Paper - Transformation of the aortic-arch system during the development of the human embryo (1922). Retrieved from https://embryology.med.unsw.edu.au/embryology/index.php/Paper_-_Transformation_of_the_aortic-arch_system_during_the_development_of_the_human_embryo_(1922)
- © Dr Mark Hill 2024, UNSW Embryology ISBN: 978 0 7334 2609 4 - UNSW CRICOS Provider Code No. 00098G
- Head
- Cardiovascular
- Historic Embryology
- Human
- Artery
- Pharyngeal Arch
- Carnegie Collection
- Carnegie Embryo 1075
- Carnegie Embryo 1121
- Carnegie Embryo 1201
- Carnegie Embryo 1201b
- Carnegie Embryo 1354
- Carnegie Embryo 1380
- Carnegie Embryo 1390
- Carnegie Embryo 163
- Carnegie Embryo 1771
- Carnegie Embryo 1878
- Carnegie Embryo 1909
- Carnegie Embryo 2053
- Carnegie Embryo 2841
- Carnegie Embryo 2937
- Carnegie Embryo 391
- Carnegie Embryo 460
- Carnegie Embryo 470
- Carnegie Embryo 492
- Carnegie Embryo 544
- Carnegie Embryo 588
- Carnegie Embryo 617
- Carnegie Embryo 721
- Carnegie Embryo 74
- Carnegie Embryo 792
- Carnegie Embryo 810
- Carnegie Embryo 826
- Carnegie Embryo 836
- Carnegie Embryo 873
- Carnegie Embryo 886
- Carnegie Embryo 940
- Carnegie Embryo 988