Magnetic Resonance Imaging
Embryology - 27 Apr 2024 ![]() ![]() ![]() |
---|
Google Translate - select your language from the list shown below (this will open a new external page) |
العربية | català | 中文 | 中國傳統的 | français | Deutsche | עִברִית | हिंदी | bahasa Indonesia | italiano | 日本語 | 한국어 | မြန်မာ | Pilipino | Polskie | português | ਪੰਜਾਬੀ ਦੇ | Română | русский | Español | Swahili | Svensk | ไทย | Türkçe | اردو | ייִדיש | Tiếng Việt These external translations are automated and may not be accurate. (More? About Translations) |
Introduction
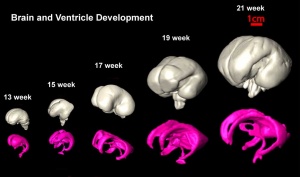
Recently there have been several groups preparing magnetic resonance imaging developmental embryo atlases of several species, including human[2], based upon imaging of different age embryos. There have been many studies of adult anatomical structures and also some of the placenta.
Magnetic Resonance Imaging (MRI) began in 1977 and uses magnetism, radio waves, and a computer to produce images either as individual slices or reconstructed to give three dimensional (3D) views of specific anatomical regions or structures.
MRI can be used in fetuses at 18 weeks gestational age or later and has been used mainly in brain and spinal diagnosis, and has also been used to investigate other abnormalities of pregnancy.
Diffusion Tensor Imaging (DTI) is a newly developed form of magnetic resonance imaging (MRI). Magnetic field variations of the MRI magnet are applied in at least six different directions generating a three dimensional shape of the diffusion pattern. This technique has be used mainly in neural imaging of white matter, due to the orientation of axon bundles and the associated directional water flow. (More? Neural Development Imaging) Computed Tomography is an alternative method of diagnostic imaging using X-rays.
- Links: Movies | Neural System - Postnatal | Computed Tomography | Category:Magnetic Resonance Imaging
About MRI
A strong magnetic field (up to 1.5 to 4 Tesla) is generated in the machine through which the body is passed (the centre of the "donut ring" seen in the image). The earth's natural magnetic field is about 0.5 Gauss compared to 15,000 Gauss (1.5 Tesla) in the MRI.
Tesla (symbol T) The SI derived unit of magnetic flux density (or magnetic inductivity) was defined in 1960 and named after Nikola Tesla.
Some Recent Findings
|
More recent papers |
---|
This table allows an automated computer search of the external PubMed database using the listed "Search term" text link.
More? References | Discussion Page | Journal Searches | 2019 References | 2020 References Search term: Fetal Magnetic Resonance Imaging | Fetal Functional Magnetic Resonance Imaging |
Older papers |
---|
These papers originally appeared in the Some Recent Findings table, but as that list grew in length have now been shuffled down to this collapsible table.
See also the Discussion Page for other references listed by year and References on this current page.
|
Human Birth
The images below are from a recent study employing new MRI equipment and methodology that allows more free access to the scanned patient compared to the original methodologies. This should allow study of complex physiological processes, such as childbirth, in relative real-time.
2010.12.07 - Press release | Birth Image | AG OMRT - Radiologie "the birth of a child in an “open” MRI (magnetic resonance imaging) scanner that allows a mother-to-be to fit fully into the machine." This birth is a vertex (head or cephalic) presentation in an occipito-anterior position. Labor stage 2 is shown with the head already within the pelvis birth canal, and lying between the maternal pubic symphysis (anterior) and the sacrum (posterior).
|
|
Species Imaging with MRI
Human
- Whole Embryo[2] "To obtain data on early human development, we used magnetic resonance (MR) imaging and episcopic fluorescence capture (EFIC) to acquire digital images of human embryos spanning the time of dynamic tissue remodeling and organogenesis (Carnegie stages 13 to 23)."
- Brain[1] "Quantitative whole brain 3D validation of tissue labeling performed on a set of 14 fetal MR scans (20.57-22.86 weeks gestational age) demonstrates that this atlas-based EM segmentation approach achieves consistently high DSC performance for the main tissue types in the fetal brain."
- Birth 2010.12.07 - Press release | Birth Image | AG OMRT - Radiologie "the birth of a child in an “open” MRI (magnetic resonance imaging) scanner that allows a mother-to-be to fit fully into the machine."
Fetal (9 month) MRI[16]
Baboon
- Brain[17] "We devised a protocol to scan pregnant baboons serially at 3 T for up to 3 h per session. Seven baboons were scanned 1-6 times, beginning as early as 56 days post-conceptional age, and as late as 185 days (term approximately 185 days). Successful scanning of the fetal baboon required careful animal preparation and anesthesia, in addition to optimization of the scanning protocol. We successfully acquired maps of relaxation times (T(1) and T(2)) and high-resolution anatomical images of the brains of fetal baboons at multiple time points during the course of gestation."
Mouse
- Mouse Mutants[18]"Using individual 3D embryo MRI histology, we identified new pituitary phenotypes in Hesx1 mutant mice. Subsequently we use advanced computational techniques to produce a whole-body embryo atlas from 6 CD-1 embryos, creating an average image with a greatly enhanced anatomical detail, particularly in CNS structures."
- A 4D atlas and morphologic database[19] "This work makes magnetic resonance microscopy of the mouse embryo and neonate broadly available with carefully annotated normative data and an extensive environment for collaborations."
Chicken
- Eye development [20] "We subsequently used the images obtained from the MRI data in order to make precise measurements of chick embryo eye surface area, volume and axial length from E4 to E10."
Xenopus
- Early embryo[21]"Here, we report on the use of microscopic magnetic resonance imaging (mMRI) to noninvasively observe mitotic cell division of early blastomeres in the optically opaque Xenopus laevis embryo."
Embryo Imaging
![]() |
![]() |
![]() |
MRI 01 | MRI 02 | MRI 03 |
- Links: Movies
Structure Imaging
Upper Limb
Human embryo week 6 (GA 8 week) and week 7 (GA 9 week).[9] Ex vivo magnetic resonance microscopy (MRM) at 7.1 T (Clin Scan, Bruker Biospin, Germany) was performed in 10 human specimens at 8 to 12 weeks of gestational age (GA). In-plane resolution was 20 μm with a slice thickness of 70 μm.
a GA 8 week Sagittal T2w - humerus | b GA 8 week Coronal T2w - forearm | c GA 9 week Coronal T2w - forearm |
---|---|---|
Shows initial ossification within the central part of the diaphysis (arrow).
Chondrified ribs (short arrow). |
Shows small ossification centers in the central parts of the radius and ulna (arrow).
The carpal (dotted white arrow) and metacarpal bones (dotted black arrow) are already visible as precartilage states. |
Shows increased size of the ossification centers in humerus (white arrowhead) and radius (black arrow).
The carpal and metacarpal bones demonstrate progressive chondrification and appear hypointense compared to the 8-week GA specimen |
Table of Ossification of the Bones of the Superior Extremity | |||
---|---|---|---|
(Days and weeks refer to the prenatal, years to the postnatal period.) | |||
Bone | Centres | Time of appearance of centre | Union of primary and secondary centres; remarks. |
Clavicle | Diaphysis | 6th week | There are two centres in the shaft, a medial and a lateral. These blend on the 45th day (Mall). Shaft and epiphysis unite between the 20th and 25th years. |
Sternal epiphysis | 18th to 20th year | ||
Scapula | Primary centres: | The chief centre appears near the lateral angle. The subcoracoid centre appears at the base of the coracoid process and also gives rise to a part of the superior margin of the glenoid fossa. The coracoid process joins the body about the age of puberty. The acromial epiphysis centres (two or three in number) fuse with one another soon after their appearance and with the spine between the 22nd and 25th years (Quain); 20th year (Wilms). The subcoracoid and the epiphysis of the coracoid process, the glenoid fossa, the inferior angle, and the vertebral margin join between the 18th and 24th years in the order mentioned (Sappey). | |
1. That of the body, the spine, and the base of the glenoid cavity. | 8th week (Mall) 1 | ||
2. Goraooid process | 1st year | ||
3. Subcoracoid | 10th to 12th year | ||
Epiphyses: | |||
Acromial epiphyses | 15th to 18th year | ||
Epiphysis of the inferior angle. | 16 to 18th year | ||
Epiphyses of the vertebral border. | 18th to 20th year | ||
Epiphyses of upper surface of coracoid. | 16th to 18th year. | ||
Epiphysis of surface of glenoid fossa. | 16th to 18th year. | ||
Humerus | Diaphysis | 6th to 7th week (Mall) | The epiphyses of the head, the tuberculum majus and the tuberculum minus (the last is inconstant) unite with one another in 4th-6th year and with the shaft in 20th-25th year. The epiphyses of the capitulum, lateral epicondyle, and trochlea unite with one another and then in the 16th-17th year join the shaft. The epiphysis of the medial epicondyle joins the shaft in the 18th year. |
Epiphyses: | |||
Head | 1st to 2d year | ||
Tuberculum majus | 2d to 3d year | ||
Tuberculum minus | 3d to 5th year | ||
Capitulum | 2d to 3d year | ||
Epioondylus med | 5th to 8th year | ||
Lateral margin of trochlea | 11th to 12th year | ||
Epicondylus lat | 12th to 14th year | ||
Radius | Diaphysis | 7th week (Mall) | The superior epiphysis and shaft unite between the 17th and 20th years. The inferior epiphysis and shaft about the 21st year (Pryor); M 21st year, F 21st-25th year (Sappey). Sometimes an epiphysis is found m the tuberosity (R. and K.) and in the styloid process (Sappey). |
Epiphyses: | |||
Carpal end | F 8th month - M 15th month (Pryor) | ||
Humeral end | 6th-7th year | ||
Ulna | Diaphysis | 7th week | The centre for the shaft of the ulna arises a few days later than that for the radius. The proximal epiphysis is united to the shaft about the 17th year; the inferior epiphysis between the 18th and 20th years; F 20th - 21st years, M 21st - 24th years (Sappey). There is sometimes an epiphysis in the styloid process (Sohwegel) and in the tip of the olecranon process (Sappey). |
Epiphyses: | |||
Carpal end | F 6th-7th year - M 7th-8th year (Pryor) | ||
Humeral end | 10th year | ||
Carpus | Os capitatum | F 3d-6th month M 4th-10th month | The navicular sometimes has two centres of ossification (Serres. Rambaud and Renault). Serres and Pryor have described two centres of ossification in the lunatum. Debierre has described two centres in the pisiform, one in a girl of eleven, the other in a boy of twelve. The OS hamatum may have a special centre for the hamular process. Pryor has found two centres in the triquetrum. Pryor (1908), describes the centres of ossification of the carpal bones as assuming shapes characteristic of each bone at an early period. |
Os hamatum | F 5th-10th month M 6th-12th month | ||
Os triquetrum | F 2d-3d year M about 3 years | ||
Os lunatum | F 3rd-4th year M about 4 years | ||
Os naviculare | F at 4 years, or early in 5th year M about 5 years | ||
Os mult. maj. | F 4th-5th year M 5th-6th year | ||
Osmult. min. | F 4th-5th year M 6th-6th year | ||
Os pisiforme | F 9th-10th year M 12th-3th year | ||
Metacarpals | Diaphyses | 9th week (Mall) | The centres for the shafts of the second and third metacarpals are the first to appear. There may be a distal epiphysis for the first metacarpal and a proximal epiphysis for the second. Pryor (1906). found the distal epiphysis of the first metacarpal in about 6 per cent, of cases. It is a family characteristic. It arises before the 4th year and unites later. Pryor found the proximal epiphysis of the second metacarpal in six out of two hundred families. It unites with the shaft between the 4th and 6th-7th year; sometimes, however, not until the 14th year. In the seal and some other animals all the metacarpals have proximal and distal epiphyses (Quain). The epiphyses join the shafts between the 15th and 20th years. There may bean independent epiphysis for the styloid process of the 5th metacarpal. The epiphysis of the metacarpal of the index finger appears first. This is followed by those of the 3d, 4th, 5th, and 1st digits. |
Proximal epiphysis of the first metacarpal | 3d year | ||
Distal epiphyses of the metacarpals | 2d year | ||
Phalanges | Diaphyses | 9th week (Mall) | |
First row | Proximal epiphyses | 1st-3rd year (Pryor) | The shafts of the phalanges of the second and third fingers are the first to show centres of ossification. The phalanges of the little finger are the last, the epiphysis in the middle finger is the first to appear. This is followed by those of the 4th, 2d, 5th, and 1st digits. |
Middle row | Diaphyses | 11th-12th week (Mall) | The centres in the shafts of this row are the last to appear. The epiphysis of the phalanx of the middle finger is the first to appear. This is followed by those of the ring, index, and little finger (Pryor). |
Proximal epiphyses | 2nd-3rd year | ||
Terminal row | Diaphyses | 7th-8th week | The terminal phalanx of the thumb is the first to show a centre of ossification in the shaft. This is the first centre of ossification in the hand. It is developed in connective tissue while the centres of the other phalanges are developed in cartilage (Mall). The epiphysis of the ungual phalanx of the thumb is followed by those of the middle, ring, index, and little fingers. The fusion of the epiphyses of the phalanges with the diaphyses takes place in the 18th-20th year. |
Proximal epiphyses | 2nd-3rd year | ||
Sesamoid bones | Ossification begins generally in the 13th - 14th years, and may not take place until after middle life (Thilenius). For table of relative frequency in the embryo and adult see p. 385. | ||
M = male F = female. | |||
Reference: Manual of Human Embryology by Franz Keibel and Franklin P. Mall (1910) Table - Upper Limb |
- Links: Limb Development | Bone Development | Bone Timeline
Placenta
MRI normal placenta different gestational ages.[22]
![]() |
Magnetic resonance angiography (MRA) of human placenta viewed from the fetal side.[23] |
- Links: Placenta Development
Adult Inner Ear
The 3D reconstructed technique was used to acquire coronal and axial images of the adult inner ear. The coronal section reconstruction was chosen since it increases visibility of the turns of the cochlea.[24]
![]() |
![]() |
Adult Skull
Adult Skull MRI | Links: | Skull Development | - MRI | ||||||||||||
---|---|---|---|---|---|---|---|---|---|---|---|---|---|---|---|
|
|
|
|
Neural
The following images are from human fixed fetal brains scanned with diffusion tensor magnetic resonance imaging.[1]
Diffusion tensor imaging (DTI) A newly developed form of magnetic resonance imaging (MRI). Magnetic field variations of the MRI magnet are applied in at least six different directions generating a three dimensional shape of the diffusion pattern. This technique can be used in neural imaging of white matter due to the orientation of axon bundles and the associated water flow. (More? Magnetic Resonance Imaging)
- Neural DTI Links: Scaled Fissures 13-21 weeks | Fissures 13-21 weeks | Brain Sylvian Fissure | Scaled Brain and Ventricles 13-21 weeks | Scaled Brain, Ventricles and Ganglia 13-21 weeks | Limbic Tract 13-19 weeks | Brain and Ventricles 13-21 weeks | Sylvian Fissure Movie | Neural System Development | Magnetic Resonance Imaging
Brain Tract
Brain Ventricles and Ganglia
Brain Fissures
Cortical surfaces for neonates at 28, 36 and 44 weeks PMA at scan with the labels overlaid.[25]
- Links: neural | Cerebrum Development
Prenatal Diagnosis
MRI can be used in fetuses at 18 weeks gestational age or later and has been used mainly in brain and spinal diagnosis, and has also been used to investigate other abnormalities of pregnancy.
- Absence of harmful effects of magnetic resonance exposure at 1.5 T in utero during the third trimester of pregnancy: a follow-up study.{{#pmid:15234454|PMID15234454"Thirty-five children between 1 and 3 years of age, and nine children between 8 and 9 years of age, that were exposed to MR during the third trimester of pregnancy, were checked for possible adverse effects in a follow-up study. Data on pregnancy and birth, the results of a neurological examination at 3 months, their medical documentary with emphasis on eye and ear functioning, and a questionnaire answered by their mothers were collected and evaluated. In five children abnormal test results were observed, that had no relation to the MR exposure. No harmful effects of prenatal MR exposure in the third trimester of pregnancy were detected in this study."
- Prenatal diagnosis of neurofibromatosis type 1: sonographic and MRI findings.[26] "Prenatal ultrasound and magnetic resonance imaging (MRI) demonstrated a large oropharyngeal tumor, and cardiac and cranial abnormalities consistent with neurofibromatosis type 1 (NF1) in a third-trimester fetus, which were confirmed on postmortem examination. Sonographic features of NF1 are generally nonspecific; MR examination provided significant additional information, facilitating prenatal diagnosis."
- In utero magnetic resonance imaging for brain and spinal abnormalities in fetuses.[27] "In the past eight years magnetic resonance imaging has been used to detect fetal abnormalities in utero at many centres around the world."
Links: Prenatal Diagnosis | Magnetic Resonance Imaging
References
- ↑ 1.0 1.1 1.2 Habas PA, Kim K, Rousseau F, Glenn OA, Barkovich AJ & Studholme C. (2010). Atlas-based segmentation of developing tissues in the human brain with quantitative validation in young fetuses. Hum Brain Mapp , 31, 1348-58. PMID: 20108226 DOI.
- ↑ 2.0 2.1 2.2 Yamada S, Samtani RR, Lee ES, Lockett E, Uwabe C, Shiota K, Anderson SA & Lo CW. (2010). Developmental atlas of the early first trimester human embryo. Dev. Dyn. , 239, 1585-95. PMID: 20503356 DOI.
- ↑ Chalouhi GE, Millischer AÉ, Mahallati H, Siauve N, Melbourne A, Grevent D, Vinit N, Heidet L, Aigrain Y, Ville Y, Blanc T & Salomon LJ. (2019). The use of fetal MRI for renal and urogenital tract anomalies. Prenat. Diagn. , , . PMID: 31736096 DOI.
- ↑ Khan S, Vasung L, Marami B, Rollins CK, Afacan O, Ortinau CM, Yang E, Warfield SK & Gholipour A. (2019). Fetal brain growth portrayed by a spatiotemporal diffusion tensor MRI atlas computed from in utero images. Neuroimage , 185, 593-608. PMID: 30172006 DOI.
- ↑ Vasung L, Charvet CJ, Shiohama T, Gagoski B, Levman J & Takahashi E. (2019). Ex vivo fetal brain MRI: Recent advances, challenges, and future directions. Neuroimage , 195, 23-37. PMID: 30905833 DOI.
- ↑ Konkel L. (2018). The Brain before Birth: Using fMRI to Explore the Secrets of Fetal Neurodevelopment. Environ. Health Perspect. , 126, 112001. PMID: 30457876 DOI.
- ↑ Nagarajan M, Sharbidre KG, Bhabad SH & Byrd SE. (2018). MR Imaging of the Fetal Face: Comprehensive Review. Radiographics , , 170142. PMID: 29652578 DOI.
- ↑ Scola E, Conte G, Palumbo G, Avignone S, Cinnante CM, Boito S, Persico N, Rizzuti T & Triulzi F. (2018). High resolution post-mortem MRI of non-fixed in situ foetal brain in the second trimester of gestation: Normal foetal brain development. Eur Radiol , 28, 363-371. PMID: 28755056 DOI.
- ↑ 9.0 9.1 Langner I, Stahnke T, Stachs O, Lindner T, Kühn JP, Kim S, Wree A & Langner S. (2016). MR microscopy of the human fetal upper extremity - a proof-of-principle study. BMC Dev. Biol. , 16, 21. PMID: 27316469 DOI.
- ↑ 10.0 10.1 Saleem SN. (2014). Fetal MRI: An approach to practice: A review. J Adv Res , 5, 507-23. PMID: 25685519 DOI.
- ↑ Boyer AC, Gonçalves LF, Lee W, Shetty A, Holman A, Yeo L & Romero R. (2013). Magnetic resonance diffusion-weighted imaging: reproducibility of regional apparent diffusion coefficients for the normal fetal brain. Ultrasound Obstet Gynecol , 41, 190-7. PMID: 22744761 DOI.
- ↑ Bamberg C, Rademacher G, Güttler F, Teichgräber U, Cremer M, Bührer C, Spies C, Hinkson L, Henrich W, Kalache KD & Dudenhausen JW. (2012). Human birth observed in real-time open magnetic resonance imaging. Am. J. Obstet. Gynecol. , 206, 505.e1-6. PMID: 22425409 DOI.
- ↑ Hibbeln JF, Shors SM & Byrd SE. (2012). MRI: is there a role in obstetrics?. Clin Obstet Gynecol , 55, 352-66. PMID: 22343250 DOI.
- ↑ Correia L, Ramos AB, Machado AI, Rosa D & Marques C. (2012). Magnetic resonance imaging and gynecological devices. Contraception , 85, 538-43. PMID: 22133659 DOI.
- ↑ Huang H, Xue R, Zhang J, Ren T, Richards LJ, Yarowsky P, Miller MI & Mori S. (2009). Anatomical characterization of human fetal brain development with diffusion tensor magnetic resonance imaging. J. Neurosci. , 29, 4263-73. PMID: 19339620 DOI.
- ↑ Hoseinian-Azghadi E, Rafat-Motavalli L & Miri-Hakimabad H. (2014). Development of a 9-months pregnant hybrid phantom and its internal dosimetry for thyroid agents. J. Radiat. Res. , 55, 730-47. PMID: 24515254 DOI.
- ↑ Liu F, Garland M, Duan Y, Stark RI, Xu D, Dong Z, Bansal R, Peterson BS & Kangarlu A. (2008). Study of the development of fetal baboon brain using magnetic resonance imaging at 3 Tesla. Neuroimage , 40, 148-59. PMID: 18155925 DOI.
- ↑ Cleary JO, Modat M, Norris FC, Price AN, Jayakody SA, Martinez-Barbera JP, Greene ND, Hawkes DJ, Ordidge RJ, Scambler PJ, Ourselin S & Lythgoe MF. (2011). Magnetic resonance virtual histology for embryos: 3D atlases for automated high-throughput phenotyping. Neuroimage , 54, 769-78. PMID: 20656039 DOI.
- ↑ Petiet AE, Kaufman MH, Goddeeris MM, Brandenburg J, Elmore SA & Johnson GA. (2008). High-resolution magnetic resonance histology of the embryonic and neonatal mouse: a 4D atlas and morphologic database. Proc. Natl. Acad. Sci. U.S.A. , 105, 12331-6. PMID: 18713865 DOI.
- ↑ Goodall N, Kisiswa L, Prashar A, Faulkner S, Tokarczuk P, Singh K, Erichsen JT, Guggenheim J, Halfter W & Wride MA. (2009). 3-Dimensional modelling of chick embryo eye development and growth using high resolution magnetic resonance imaging. Exp. Eye Res. , 89, 511-21. PMID: 19540232 DOI.
- ↑ Papan C, Boulat B, Velan SS, Fraser SE & Jacobs RE. (2006). Time-lapse tracing of mitotic cell divisions in the early Xenopus embryo using microscopic MRI. Dev. Dyn. , 235, 3059-62. PMID: 16958098 DOI.
- ↑ Varghese B, Singh N, George RA & Gilvaz S. (2013). Magnetic resonance imaging of placenta accreta. Indian J Radiol Imaging , 23, 379-85. PMID: 24604945 DOI.
- ↑ Rasmussen AS, Lauridsen H, Laustsen C, Jensen BG, Pedersen SF, Uhrenholt L, Boel LW, Uldbjerg N, Wang T & Pedersen M. (2010). High-resolution ex vivo magnetic resonance angiography: a feasibility study on biological and medical tissues. BMC Physiol. , 10, 3. PMID: 20226038 DOI.
- ↑ Pochini Sobrinho F, Lazarini PR, Yoo HJ, Abreu Júnior Ld & Meira Ade S. (2009). A method for measuring the length of the cochlea through magnetic resonance imaging. Braz J Otorhinolaryngol , 75, 261-7. PMID: 19575114
- ↑ Makropoulos A, Aljabar P, Wright R, Hüning B, Merchant N, Arichi T, Tusor N, Hajnal JV, Edwards AD, Counsell SJ & Rueckert D. (2016). Regional growth and atlasing of the developing human brain. Neuroimage , 125, 456-478. PMID: 26499811 DOI.
- ↑ McEwing RL, Joelle R, Mohlo M, Bernard JP, Hillion Y & Ville Y. (2006). Prenatal diagnosis of neurofibromatosis type 1: sonographic and MRI findings. Prenat. Diagn. , 26, 1110-4. PMID: 16981221 DOI.
- ↑ Griffiths PD, Paley MN, Widjaja E, Taylor C & Whitby EH. (2005). In utero magnetic resonance imaging for brain and spinal abnormalities in fetuses. BMJ , 331, 562-5. PMID: 16150769 DOI.
Reviews
Vasung L, Charvet CJ, Shiohama T, Gagoski B, Levman J & Takahashi E. (2019). Ex vivo fetal brain MRI: Recent advances, challenges, and future directions. Neuroimage , 195, 23-37. PMID: 30905833 DOI.
Wright C, Sibley CP & Baker PN. (2010). The role of fetal magnetic resonance imaging. Arch. Dis. Child. Fetal Neonatal Ed. , 95, F137-41. PMID: 19208672 DOI.
Elsayes KM, Trout AT, Friedkin AM, Liu PS, Bude RO, Platt JF & Menias CO. (2009). Imaging of the placenta: a multimodality pictorial review. Radiographics , 29, 1371-91. PMID: 19755601 DOI.
Chung R, Kasprian G, Brugger PC & Prayer D. (2009). The current state and future of fetal imaging. Clin Perinatol , 36, 685-99. PMID: 19732621 DOI.
Reddy UM, Filly RA & Copel JA. (2008). Prenatal imaging: ultrasonography and magnetic resonance imaging. Obstet Gynecol , 112, 145-57. PMID: 18591320 DOI.
Laifer-Narin S, Budorick NE, Simpson LL & Platt LD. (2007). Fetal magnetic resonance imaging: a review. Curr. Opin. Obstet. Gynecol. , 19, 151-6. PMID: 17353684 DOI.
Articles
Nemec SF, Kasprian G, Brugger PC, Bettelheim D, Amann G, Nemec U, Rotmensch S, Graham JM, Rimoin DL, Lachman RS & Prayer D. (2011). Abnormalities of the upper extremities on fetal magnetic resonance imaging. Ultrasound Obstet Gynecol , 38, 559-67. PMID: 21308835 DOI.
Cleary JO, Modat M, Norris FC, Price AN, Jayakody SA, Martinez-Barbera JP, Greene ND, Hawkes DJ, Ordidge RJ, Scambler PJ, Ourselin S & Lythgoe MF. (2011). Magnetic resonance virtual histology for embryos: 3D atlases for automated high-throughput phenotyping. Neuroimage , 54, 769-78. PMID: 20656039 DOI.
Vincent K, Moore J, Kennedy S & Tracey I. (2009). Blood oxygenation level dependent functional magnetic resonance imaging: current and potential uses in obstetrics and gynaecology. BJOG , 116, 240-6. PMID: 19076956 DOI.
Sarikouch S, Schaeffler R, Körperich H, Dongas A, Haas NA & Beerbaum P. (2009). Cardiovascular magnetic resonance imaging for intensive care infants: safe and effective?. Pediatr Cardiol , 30, 146-52. PMID: 18709400 DOI.
Schneider JE, Böse J, Bamforth SD, Gruber AD, Broadbent C, Clarke K, Neubauer S, Lengeling A & Bhattacharya S. (2004). Identification of cardiac malformations in mice lacking Ptdsr using a novel high-throughput magnetic resonance imaging technique. BMC Dev. Biol. , 4, 16. PMID: 15615595 DOI.
Search PubMed
Search Pubmed: Embryo Magnetic Resonance Imaging | Magnetic Resonance Imaging |
NCBI - Policies and Guidelines | PubMed | Help:Reference Tutorial
Terms
- Balanced gradient echo - a sequence image useful in detection of the edges of organs and lesions. Does not show the organ detail, but enhances the edges.
- Diffusion-weighted image - (DWI) useful in imaging the neural (brain, CSF) and renal (kidney, shape and size). Brain image is age-dependent and symmetrical. Neural diagnostically for small haemorrhages, infarcts, and infection damage.
- T1 weighted image - (T1W, spin-lattice relaxation time) a sequence image with low spatial resolution and useful in detection (bright) imaging of the liver, thyroid, fat and meconium.
- T2 weighted image - (T2W) a sequence image useful in providing the most detailed fetal anatomy.
External Links
External Links Notice - The dynamic nature of the internet may mean that some of these listed links may no longer function. If the link no longer works search the web with the link text or name. Links to any external commercial sites are provided for information purposes only and should never be considered an endorsement. UNSW Embryology is provided as an educational resource with no clinical information or commercial affiliation.
- DTI Brain parameter atlas
- Brookhaven National Laboratory New MicroMRI Facility Expands Lab's Brain-Imaging Capabilities
- 3-D MRI Digital Atlas Database of an Adult C57BL/6J Mouse Brain
- Related internal links: Mouse Development | Neural System Development
- Beth Israel Deaconess Medical Center Atlas of Fetal MRI - head and neck | brain | face | spine | chest | abdomen | genitourinary | extremities
- MR open access image processing - toolkit version 1.9.05
Glossary Links
- Glossary: A | B | C | D | E | F | G | H | I | J | K | L | M | N | O | P | Q | R | S | T | U | V | W | X | Y | Z | Numbers | Symbols | Term Link
Cite this page: Hill, M.A. (2024, April 27) Embryology Magnetic Resonance Imaging. Retrieved from https://embryology.med.unsw.edu.au/embryology/index.php/Magnetic_Resonance_Imaging
- © Dr Mark Hill 2024, UNSW Embryology ISBN: 978 0 7334 2609 4 - UNSW CRICOS Provider Code No. 00098G