2014 Group Project 5
2014 Student Projects | ||||
---|---|---|---|---|
2014 Student Projects: Group 1 | Group 2 | Group 3 | Group 4 | Group 5 | Group 6 | Group 7 | Group 8 | ||||
The Group assessment for 2014 will be an online project on Fetal Development of a specific System.
This page is an undergraduate science embryology student and may contain inaccuracies in either description or acknowledgements. |
Integumentary
Introduction
This page concerns the development of the integumentary system in the fetal stage of development, particularly its organs i.e. the skin, glands, hair, teeth, and nails. It explores the mechanism of development as well as the timeline of development. This page also outlines some recent findings on the development of the integumentary system, as well as historic findings. Finally, this page also explores some of the congenital abnormalities of the integumentary system, its mechanism or pathogenesis, clinical manifestations, and how they are treated or managed.
Objectives |
---|
|
Development Overview
Skin
The skin consists of 2 layers: the outer layer (epidermis) and a deeper connective tissue layer (dermis)[1].
- The epidermis is derived from the ectoderm. Initially it exists as only a single layer of ectodermal cells at 7-8 days of gestation[2]. However, by about 13-14 weeks after gestation, a 3- layered structure of fetal epidermis exists[3]- consisting of the stratum basale, 1 or 2 intermediate layers and the periderm[4]. The peridermal cells eventually become desquamated and form part of the vernix cervix.
- The somatic mesoderm is the embryonic origin of the dermis. The mesoderm of the dermatones of the body, also contribute to the development of the dermis. Specifically though, in the head and neck region of the body, the dermis is derived from neural crest cells[7].
- The dermis is initially composed of just mesenchymal cells- loosely aggregated mesodermal cells. These mesenchymal cells later develop into fibroblasts- which function to secrete collagen and lay-down elastic fibers into the extracellular matrix[7].
3 other specialised cells of the epidermis also exists[7] - these include melanoblasts, Langherhan cells and Merkel cells.
- Melanoblasts- are derived from neural crest cells that have migrated into the stratum basale. Mid-pregnancy, melanosomes are observed, differentiating the melanoblasts into melanocytes[7].
- Langheran cells- are derived from bone marrow (originally form mesoderm) and migrate into the epidermis[7]. They have the function of antigen presentation.
- Merkel cells- still have an uncertain origin. They have a function related to mechanoreception.
Week | Description | Phase Diagram |
---|---|---|
Weeks 6-8 | In an electron micrograph study of the epidermis[3], the periderm and and basal layer of the developing skin was observed. The basal cell keratins K5 and K14 were also observed from 8 weeks onwards[3] | ![]() |
Weeks 7-9 | In an electron micrograph study of the epidermis at weeks 7-9 of development, the stratified three-layer structure of the epidermis was observed[3]; with the stratum intermedium forming between the basal and periderm layers . Kertain filaments, such as K8 and K19 have been encircled- they feature during fetal skin development but are absent in the adult epidermis. | ![]() |
Weeks 14-16 | By week 14, the basal layer, the intermediate layer/s and the periderm 3-layered structure can be observed in the fetus[3]. By week 14, K17 can also be found in the basal and intermediate layers of the epidermis (In adult skin, K17 is not observed) [2]. Developing blood vessels were observed at the end of week 16[2]. | ![]() |
Weeks 20-22 | By week 20, hair follicles can be already be seen in the epidermis. The total number of intermediate layers has also increased[2]. In an electron micrograph study at week 22 of development[3], kertanised epidermis was analysed. It was observed that glycogen was abundantly present throughout all epidermal layers. (The included arrows, highlight the keratin filament bundles, which are now organised and peripherally placed.) | ![]() |
Adult | In adult skin- a greater diversity of cells can be seen as more cells differentiate. Basal, spinous, granular and cornified cells are all example of such[4][6]. The fetal extra-cellular matrix also differs from that of the adult- mainly in terms of the collagen type[8]. and amount of glycosaminoglycans present[9]. | ![]() |
Hair
Hair originates from the ectoderm. At about 12 weeks, it is believed that specific signals from the dermis are released- signaling for the induction hair follicle formation [10][11]
Stage | Weeks | Description |
---|---|---|
(a) Undifferentiated Epithelium | Weeks 8-12 | Through reciprocal interactions and ‘first dermal signaling’, cells from the stratum basale grow into the underlying dermis. The signaling pathway, however, has not been fully identified[5] |
(b) Placode | Weeks 12-14 | The ‘first dermal signals’ influence epithelial cells to develop a placode- a thickening of the columnar cells. It is theorised that varying intrinsic dermal signals lead to the expression of various placodes and consequently, the differences in the expression of hair thickness/size throughout the body[12]. The specific combination of promoter and repressor activators for hair development, is also theorised to characterise for the regional differences in eventual hair expression[13] |
(c) Germ | Weeks 13-16 | WNT Signalling is believed to have a role in the induction of the dermal condesate[5]. Platelet-derived growth factor-A molecules from the placode, also contributes to the induction of the dermal condensate. The development of the dermal condensate helps further induce the downward growth of the placode.
Through secreted proteins such as Sonic Hedgehog, the placode continues to proliferate and enclose the dermal condensate. This eventually forms a deep, club-shaped hair bud, with an invaginated dermal papillae [5]. These dermal papillae are rapidly infiltrated by blood vessels and nerve endings. |
(d) Peg | Weeks 19-21 | Sonic Hedgehog and the induction of a ‘secondary dermal signal’ (characterisation unknown) leads to a significant down-growth and proliferation of the follilular epithelium[14].
In this stage, it is also believed that the polarity of the hair follicle (the angle at which hair-follicles grow in relation to skin) and the architecture of the hair follicle itself (straight hair, wavy hair, etc) is regulated in part by Sonic Hedgehog and TGF-a signaling respectively[5]. |
(e) Bulbous Peg | Weeks 23-28 | This stage is characterised by the appearance of the hair follicle bulb. Further and significant differentiation of the inner root sheeth and the hair shaft also characterises this stage[5].
The epithelial cells within the hair bulb, begin to differentiate into the germinal matrix – which grow, proliferate and keratinise to form the hair shaft and internal root sheet[5]. Other epithelial cells outside of the hair bud, form the external hair sheeth. Mesodermal cells of the dermis that surround the invaginating hair follicle form the dermal root sheeth and the arrecrtor pili muscles for hairs. Proteins such as Notch1 are believed to help regulate the phenotype of keratinocytes as they differentiate[15]. |
Lanugo Hair[1]
- Appear at the end of week 12
- Abundant from weeks 17-20
- Shed 4 weeks before birth [16]
- Lanugo Hairs are the first fetal hairs. They are characterised by their soft, fine and unpigmented nature.
- Lanugo Hairs have a role in keeping the vernix caseosa intact to the fetus[1]
Nail
Together, fingernails and toenails are modifications of the epidermis which are derived from the same embryonic origin, the ectoderm. [17]
Week | Event |
---|---|
Week 9 | The primitive finger nail beings to from. Preceding the morphological development, molecular signalling molecules being patterning the ectodermal layer. Signal molecules such as Bone Morphogentic Proteins (BMPs) allow communication between tissue layers and are involved in the initiating development of the nail. [18] |
Week 10 | The primary nail field is establish, marked by a localised thickening of the epithelium. This primary nail fields initial from on the ventral surface of the digits and are repositioned to the dorsal side during development. [1] The LIM-homeodomain protein (Lmx1b) is a signalling molecule involved in this process, it's localised expression allows the dorsal-vetral limb axis to be established. A mutation in the gene coding for Lmx1b correlates with abnormal development of the nail and other bony structures. [19] |
Week 11 | On the lateral edges of the primary nail field, ectodermal cells proliferate to from the shallow lateral nail folds. Similarly proliferation of the cells on the proximal end of the nail field gives rise to the deeper proximal nail fold. The nail field now appears as a distinct region on the digits. [17]. In addition, the distal ridges of nail bed keratinise. |
Week 13 | Seen in cross-section, the early nail matrix begins to from, this marked region with in the proximal nail fold which undergoes localised cornification. The nail plate grows from the nail matrix as kertaised cells are flattened and compacted into dense nail tissue. |
Week 14 | The primitive toe nails being to from. This event usually occurs 4 weeks after development of the finger nails. The differential timing of these events is established by signalling molecules that establish the rostro-cauda sequence of development in the embryo and fetus. |
Week 20 | Nail plate begins to grow over the nail bed from the proximal nail matrix towards the distal direction. |
Week 24 | Free nail plate is visible to the naked eye. Initially the developing nail is covered by a thin layer of epidermis known as the eponychium (corneal layer of epidermis). At this stage in fetal development the eponychium declines, the cuticle remains over the proximal nail plate. Below the free end of the nail, epidermal cells aggregate to form the mass known as the hyponychium[1]. |
Week 32-36 | The finger nails and toe nails respectively reach the tips of the digits and the toes. |
Glands
Gland Type | Description | Image |
---|---|---|
Sebaceous Glands | Sebaceous glands develop from the epithelial wall of the hair follicle. They secrete the vernix caseosa
Vernix caseosa is a material secreted by sebaceous glands in the foetus in the last trimester of development (Week 21)[20] and is characterised by it’s cheese-like appearance around the neonate at birth. The functions of vernix caseosa include: |
![]() Vernix caseosa on a neonate.[23] |
Mammary Glands | Mammary glands develop from the mammary ridge- a downgrowth of the epidermis (ectoderm) into the underlying dermis (mesoderm). This occurs at about week 6 of development. Mammary glands first develop into primary mammary buds[1], which successively grow in length and complexity. Prior to puberty, the mammary glands are anatomically indistinguishable.[24] | |
Sweat Glands |
There are two major kinds of sweat glands present in humans, both of which develop from downgrowths of the epidermis into the underlying dermis. Sweat glands have been histologically identified in studies from week 21 of development. They begin as cellular buds[1], which proliferate as solid, cylindrical down growths into mesenchyme. Central cells degenerate to form a lumen, while the terminal region coils to eventually form the body of the gland. As fetal development continues, peripheral cells eventually differentiate into secretory and myoepithelial cells[1]. Eccrine Sweat Glands
Apocrine Sweat Glands |
Teeth
The ectoderm and the associated underlying layer of neural crest cells, are the origin for teeth development. Teeth have the main function of processing food, but also frequently serve other functions in relation to defense, display of dominance and phonetic articulation in humans [26].
Stage | Week | Description |
---|---|---|
(A) Lamina | Week 6 | As the oral ectoderm grows and proliferates, it closely interacts and has a downward movement into the underlying neural crest ectomesenchyme[26]. In the Lamina stage, teeth may grow only within the epithelium. This leads to the formation of the dental lamina. Morphological differences between the individual teeth arise due to the different expression of odontogenic genes that encode for different transcription factors that regulate the synthesis of various signaling factors[27]. |
(B) Placode | Week 7 | The dental lamina and the dental placodes arise, due to specific signals from adjacent epithelial cells. These dental placodes secrete molecules from all four growth and transcription factor families (BMPs, FGFs, SHH and WNTs) [26] to induce the expression of many genes in the mesenchyme and form tooth buds. |
(C) Bud | Week 8 | The bud stage is characterised by the appearance of a tooth blastema and a highly un-organised arrangement of cells[26]. Tooth buds are formed, as the epithelium cells interact with the messenchyme. This occurs at the sides of the dental placodes[26]. These tooth buds, later form and develop into enamel organs.
Also, as opposed to the earlier Lamina stage, in the Bud stage of tooth development, the odontogenic potential is lost from the epithelium and teeth may now only grow within the ectomesenchyme[28]. |
(D) Cap | Week 11 | With further differential proliferation and epithelial infolding, the bud takes upon the shape of an inverted cap[26]. Mesenchymal cells secrete various extracellular molecules that increase the concentration of growth factors- inducing shape changes and cellular differentiation for the developing tooth[26]. |
(E) Bell | Week 14 | The buds further develop and refold once again- this time forming an overall bell-shaped appearance[26]. The Bell stage of tooth development is characterised by the events of crown morphogenesis and cytodifferentiation[29].
Also, during this stage, the cells differentiate in situ, and the crown takes its final shape[26]. The dental papilla, which forms from the neural crest cells that underlie the enamel organs [30] eventually give rise to the dental pulp and odontoblasts- which produce predentin and dentin, in the adult body. With further development and immediate predentine deposition[30], these cells then take a columnar shape and differentiate into ameloblasts that start synthesizing and depositing enamel. |
Video
<html5media height="300" width="400">https://www.youtube.com/watch?v=nRH8M-arC58</html5media>
Current Research
|
|


|
|
More Recent Papers |
---|
<pubmed>23826487</pubmed> <pubmed>22342389</pubmed> <pubmed>24911066</pubmed> <pubmed>25143675</pubmed> <pubmed>23271751</pubmed> <pubmed>25249463</pubmed> <pubmed>23097355</pubmed> |
Historic Findings
Knowledge of the Integumentary expands in conjunction with technological developments that allow observation of microscopic structures. Historically animal models have been used to map the stages in the development of the fetal integumentary system.
Skin
- The fetal development of the skin has been investigated by in a targeted method by research scientists at large. In addition to this practising medical professionals ; both physicians and surgeons have had a significant contribution to our understanding of normal and abnormal fetal development.
- 1900 - Bardeen used the pig animal model to study the histogenesis of the dermomyotomes and nervous appratus. Bardeen and other scientists established that the human skin results from the union of the epithelial material derived from the ectoderm (epidermis) and the connective tissue origination from the mesoderm (dermis). [34]
Hair
The study of hair follicle development was primarily motivated by the need to understand abnormalities in development.
- 1958 - German dermatologist, Pinkus studied fetal hair follicle development using light microscopy techniques. Based on his observation he was able to propose four primary stages of hair follicle development: pre-germ, hair-germ, hair-peg and bulbous. [35] [36]
- 1959 - Pinkus expanded his study of the hair follicle the development of the hair follicle in man, especially the infundibulum and the connective tissue part.
- 1968 - Breathnach and Smith conducted through investigations into the phases of early fetal development. The light microscope has been the greatest tool in the study of human hair follicle development. Electron microscopy was not commonly used until recently and so little was known about the ultrastructure of the skin and its appendages. In their 1968 study they focused primarily on the development of the
- Breathnach with a number of other researches then expanded their studies looking at the and understand the peripheral nerves, the sweat duct and nail development were also explored and the interrelationship of cells at particular foetal stages was deduced. [37] These studies have provided information on the differentiation of cells and tissue of some functional importance and underline the role of cells and tissues.
- 1968 - Robins and Breathnatch investigated the development on the sebacious and apocrine swelling in the skin, which where closely associated with development of the hair follicle. They observed differentiation of desmosomes and development of cytoplasmic contents of the cell.
- 1969 - Chase and Eaton investigated fetal hair follicle development. Through their work they were able to stage the process. Hair follicle development begins with downwards growth fromt he level fo th dermis. Development begins with the downwards growth of the follicle structure from the level of the dermis. The follicle is a processes during the quiecent phase thought he adipose layer during gowth and differntiation. They also established that upward movement of hair inovlves the addition of next cells from the matrix of the follicle and an enlargement of each cell. Furthermore their research also showed that the epidermal and dermal layers were dynamic and interacting with each other. The most significant developments in the understanding of hair follicle development came from studies investigating the differentiation pattern of cells as the follicle develops. [37] [38] They were able to understand the cycling nature of hair follicle growth following development.
Determined that the hair fibre finally breaks through the epidermis and appres about the skin at around 19-21 weeks of development. The lanugo (foetal) hair is extremely fine with no medulla and a tip free of pigment.
Nail
Microscopy and staining techniques have been essential tools in study of nail development, anatomy and physiology. Thus, as such technology advances scientists have been able to uncover the morphological details of the nail and understand the changes that occur during nail development. A review of the history allows us to recognize the major contributor to this field. History of research on nail development also reveals the interesting debate on the origins of keratinized cells which migrate to form the nail palate in weeks 20-24 of fetal development.
- German scientists Unna and Pinkus were both highly reputable dermatologists who conducted a great deal of research on the structure and development of the skin and associated structures. ([39] [40])
- 1883 - Unna published a chapter on the anatomy and development of the human nail in the German textbook "Ziemsssen's Handbuch der Speciell Pathologie und Therapie" - Ziemsssen 's manual of special pathology and therapy. He provided some of the earliest insight into the development of the nail.
- 1927 - Pinkus conducted his investigations into fetal nail development. His findings were published in the "Handbuch Der Haut und Geschlechtskrankeiten” - Manual of Skin and Venerail Disease. Like Unna, these findings primarily focused on determining the cell types and structural feature
- Based on their findings both scientists proposed that the highly vascular nail matrix in the proximal nail fold gives rise to the nail plate during normal fetal development. [41]
- 1954 - Barton and Lewis conducted further investigations on the microscopic features of the fetal and mature nail and surrounding soft tissue. Lewis challenged and developed the model proposed by Unna and Pinkus, suggesting the "tri-partite" origin of the nail plate from three distinct regions with in the proximal region of the nail (1) the proximal nail fold (2) the matrix and (3) bed. [41]
- Prior to the 1900’s it was difficulty in obtaining normal fetal specimens. Furthermore, the histological staining techniques used to prepare slides of nail tissue often damage the specimen and little detail could be seen during the microscopic examinations. Barton and Lewis conducted this investigation in hopes that a better understanding of the normal developmental anatomy and physiology would assist physicians in diagnosing and treating abnormalities. [42]
- 1959 - Samman carried out an investigation on the blood supply of the human toe nail. It was thought that a deeper understanding of morphology and distribution of nail vasculature might allows us to infer the mechanism of nail plate genesis. Samman found capillary loops through out the nail fold and concluded that this supports the theory of multiple sites giving rise to the nail plate. He also noted that in the case of damage to the the terminal nail plate blood supply to the nail bed is increased. [43]
- 1963 - Zaias conducted his study on the embryology of the human nail. Primarily though light microscopy techniques, Zaias was able to build on our understanding of finer changes in the structure of the nail. He proposed a timeline highlighting the morphological hallmarks in each stage of normal nail development. [44] A number of follow up studies were conducted using a primate model.
The current understanding of fetal nail development is a summation contributions of many researches in the international scientific community. Today research continues, technology allows exploration beyond macroscopic and microscopic morphological changes in the fetal nail. Now the aim is to understand the molecular signalling with in the epidermal cells which drives this process.
Teeth
[45] -- <pubmed>5267156</pubmed>
Abnormalities
Aplasia Cutis Congenita
Aplasia cutis congenita (ACC) is a rare skin abnormality, characterised by the absence of all layers of the skin. It is most common to occur on the scalp (70%), specially the vertex. In severe cases, the defect can go as deep as the bone or the dura. Other sites of ACC include the skin of the limb regions. “ACC occurs in approximately 1 in 10000 live births, with a female-to-male ratio of 7:5.” The specific aetiologic agent for ACC is still unknown. It has been suggested to be genetic and/or environmental. The damage to the vertex is suggested to be the result of the biomechanical stretch at this area when the fetal brain is growing.[46]
Presently, ACC is managed via conservative treatments or surgical treatments. Conservative treatments refer to basic wound treatments and preventing infection with the use dressings and antibiotics. Surgical treatments, specifically scalp reconstruction procedures, aim to reconstruct the damage to the skin through skin grafts, local scalp flaps, and pericardial scalp flaps. Large defects are often treated using surgical treatments.[47]
Dystrophic Epidermolysis Bullosa
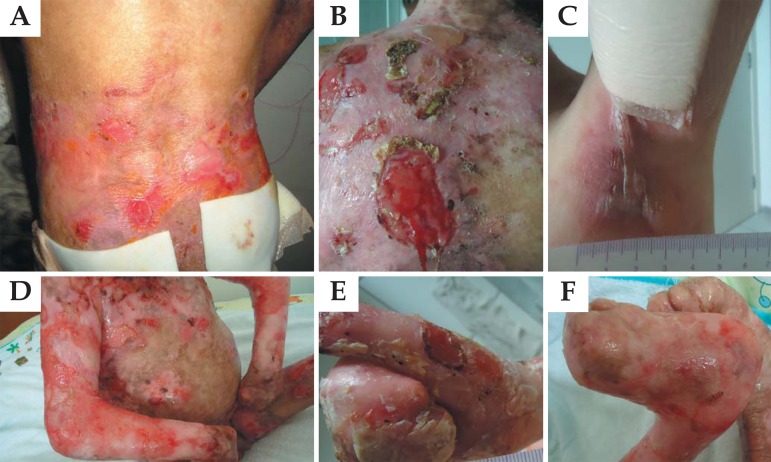
Dystrophic Epidermolysis Bullosa (DEB), a type of epidermolysis bullosa, is a genetic disease of the skin, usually present at birth or at an early age. Currently, around 400,000 - 500,000 people are affected with the disease[49]. It is characterised by the fragility of the skin[49], where it blisters upon minimal trauma and scars[50], usually at the extremities[49]. It is caused by a mutation in collagen VII gene (COL7A1)[50][51], which is responsible for the the formation of anchoring fibrils[50]. Anchoring fibrils are responsible for dermal-epidermal adherence[50], that is why it’s loss of function results to blistering of the skin. In some cases, even teeth and nails are affected. Teeth of patients with DEB have enamel defects and when combined with poor oral hygiene, it may lead to decay. Nails of DEB patients are often dystrophic and will eventually be lost.[49]
There are currently no known cures for DEB; however there are techniques to manage the clinical manifestations of the disease, which include:
- wound care[50]
- preventing factors that may cause blistering[50]
- using aqueous disinfectants - highly effective[50]
- dental care[49]:
- -use of topical fluoride
- -careful prophylaxis
- -use of topical antibiotics to prevent secondary infections[51]
One study is currently exploring the potential of protein therapy as a treatment for DEB. Their results show that intradermal injection of recombinant human collagen 7 in mice with DEB led to “restoration of C7 and anchoring fibrils.”[52] Other techniques that aim to restore C7 include:
- bone marrow transplant- improved blistering in mice specimen and increase survival rates[51]
- hematopoietic cell transplant (HCT)- increased deposition of C7 in injured skin[51]
Congenital Alopecia Areata
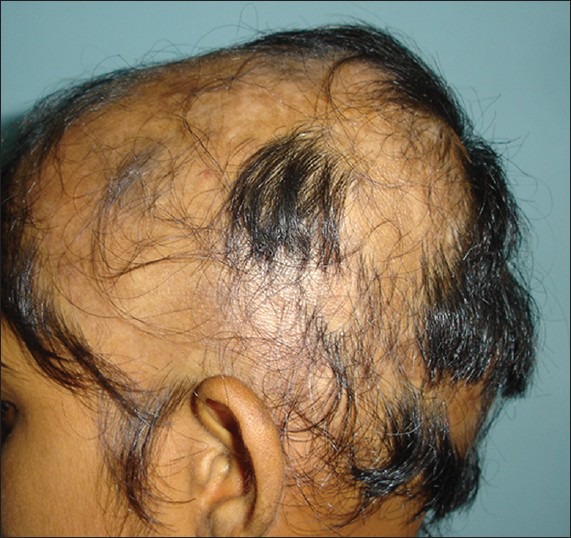
Alopecia areata (AA) is an abnormality of the hair affecting anagen hair follicles, characterised by well-demarcated patches of hair loss. It is non-scarring and can occur on the scalp and/or the body. 90% of AA cases occur on the scalp. 5%-10% of patients with AA lose all hair on their scalp; this is called alopecia totalis. While others lose all of their body hair, this is called alopecia universalis. [54] Its pathogenesis is considered to be both genetic and autoimmune. There is an abnormality with the genes related to the immune system and to the hair follicles. And histopathology shows signs of lymphatic infiltration of the hair follicles and the loss of these scalp lymphocytes allow hair follicles to recover.[55] High frequencies of catagen and telogen hair follicles are also present in areas affected by AA.[54]
There is currently no cure for AA. There are several treatments to combat AA but none of these have led to remission of the disease, the most effective being corticosteroids and topical immunotherapy.[54] A new method of treating alopecia areata is currently being studied. Transepidermal drug delivery (TED) is a new treatment that functions by creating micro-channels in the epidermis. By doing so, drug delivery to the skin is improved. This treatment was highly effective and had lower rates of side effects, e.g. pain, compared to previous treatments.[56]
Harlequin Ichthyosis

Congenital ichthyosis is an autosomal recessive disease of the skin, characterised by visible and excessive scaling of the skin and hyperkeratosis, i.e. thickening of stratum corneum layer of the epidermis and in some cases, hypohidrosis, i.e. the lack of ability to sweat. [58] Harlequin ichthyosis (HI) occurs only in 1 in 1,000,000 babies. It is life-threatening in the first few weeks and/or months of the neonate.[58] The thick skin can restrict movement of the baby and sometimes constrict extremities and lead to necrosis then autoamputation.[59] Babies with HI are also characterised by bilateral ectropion (everted eyelids), eclabium (everted lips), and underdeveloped nose.[59] In 50% of HI cases, respiratory failure is often the cause of death.[60] This disease is caused by a nonsense mutation in the ATP-binding-cassette A12 (ABCA12) gene, which is responsible for encoding a lipid transporter essential for the regulation of lamellar bodies. [58][59][60]
There is currently no known cure for this disease. Management techniques include:
- Monitoring in neonatal intensive care units.
- -Temperature within the incubator is controlled to avoid fluctuation in body temperature and to stop sweating. [58]
- Mechanical removal of excess scales from the skin [58]
- Bathing to remove excess scales from the skin[58]
- Topical therapy - to reduce hyperkeratosis. [58][60]
- Use of oral retinoids - known to have high rates of survival.[60]
Hypohidrotic Ectodermal Dysplasia

Hypohidrotic ectodermal dysplasia (HED) is the most of all ectodermal dysplasias, caused by an abnormality in the development of ectodermal tissues, which inlude skin, hair, teeth, sweat glands, and nails.[62][63] Patients with ectodermal dysplasia often have sparse hair and oligodontia, which is a condition where teeth are missing and are poorly developed.[62][63] Sweating is a very important function in the body in terms of thermoregulation. HED is mainly characterised by hypohidrosis due to the lack of sweat glands in the skin, which could lead to hyperpyrexia and sometimes death. In neonates, the mortality rate of HED reaches up to 30%, with the first year of life having the highest risk. [62] HED is caused by a genetic abnormality of the ectodysplasin A gene (EDA) and passed on by X-linked inheritance. The mutations of this gene results in the poor sweating ability or none at all in a person. The effects of this abnormality is usually more severe in males than in females. [64][63]
There is currently no pharmacological therapies for HED but there are methods applied to prevent the disease from aggravating. Neonates with HED are placed in incubators and monitored to prevent them from overheating. Management of this disease gets easier as the patient ages. Adults with HED can control their thermoregulation by staying in cool environments or drinking cold drinks to lower the body temperature. Currently, there are studies that aim to find a cure for this abnormality, e.g. gene replacement therapy in animal models.[63]
More Abnormalities |
---|
References
- ↑ 1.00 1.01 1.02 1.03 1.04 1.05 1.06 1.07 1.08 1.09 1.10 1.11 Moore, K.L., Persaud, T.V.N. & Torchia, M.G. (2011). The developing human: clinically oriented embryology (9th ed.). Philadelphia: Saunders.
- ↑ 2.0 2.1 2.2 2.3 <pubmed>19701759</pubmed>
- ↑ 3.0 3.1 3.2 3.3 3.4 3.5 <pubmed>2413039</pubmed>
- ↑ 4.0 4.1 4.2 <pubmed>168272</pubmed>
- ↑ 5.0 5.1 5.2 5.3 5.4 5.5 5.6 <pubmed>11841536</pubmed>
- ↑ 6.0 6.1 <pubmed>17039717</pubmed>
- ↑ 7.0 7.1 7.2 7.3 7.4 Dudek, R.W. (2011). BRS Embryology (5th ed.). Lippincott Williams & Wilkins
- ↑ <pubmed>8292556</pubmed>
- ↑ <pubmed>2027330</pubmed>
- ↑ <pubmed>1566372</pubmed>
- ↑ <pubmed>20590427</pubmed>
- ↑ <pubmed>10529418</pubmed>
- ↑ <pubmed>10431226</pubmed>
- ↑ <pubmed>9768360</pubmed>
- ↑ <pubmed>10804183</pubmed>
- ↑ Cite this page: Hill, M.A. (2014) Embryology 2009 Lecture 18. Retrieved October 23, 2014, from https://php.med.unsw.edu.au/embryology/index.php?title=2009_Lecture_18
- ↑ 17.0 17.1 Pansky, B. (1982). Review of Medical Embryology. Embryome Sciences, Inc 1301 Harbor Bay Parkway, Alameda, CA, 94502
- ↑ <pubmed>21387539</pubmed>
- ↑ <pubmed>9590288</pubmed>
- ↑ 20.0 20.1 20.2 <pubmed>15830002</pubmed>
- ↑ 21.0 21.1 <pubmed>21504444</pubmed>
- ↑ 22.0 22.1 22.2 22.3 <pubmed>19881987</pubmed>
- ↑ Image source: JazlynRoseVernixByPhilKonstantin.jpg http://en.wikipedia.org/wiki/File:JazlynRoseVernixByPhilKonstantin.jpg
- ↑ 24.0 24.1 24.2 <pubmed>23720046</pubmed>
- ↑ 25.0 25.1 Bolognia, J.L., Jorizzo, J.L. & Schaffer J.V. (2012). Dermatology (3rd ed.). Elsevier Limited.
- ↑ 26.0 26.1 26.2 26.3 26.4 26.5 26.6 26.7 26.8 <pubmed>19266065</pubmed>
- ↑ <pubmed>7626420</pubmed>
- ↑ <pubmed>3478009 </pubmed>
- ↑ <pubmed>1725872</pubmed>
- ↑ 30.0 30.1 <pubmed>3250849</pubmed>
- ↑ <pubmed>23377137</pubmed>
- ↑ 32.0 32.1 <pubmed>25066162</pubmed>
- ↑ <pubmed>22804461</pubmed>
- ↑ http://journals.lww.com/plasreconsurg/Citation/1949/07000/CLINICAL_ASPECTS_OF_EMBRYOLOGICAL_SKIN.8.aspx Bardeen, C. R. (1900). The development of the musculature of the body wall in the pig, including its histogenesis and its relations to the myotomes and to the skeletal and nervous apparatus. Johns Hopkins Hosp. Rep, 9, 367-399.
- ↑ Pinkus, H. (1958). Embryology of hair. The biology of .air growth, 1-32.
- ↑ <pubmed>14433089</pubmed>
- ↑ 37.0 37.1 <pubmed>5656140</pubmed>
- ↑ <pubmed>4097391</pubmed>
- ↑ <pubmed>12581143</pubmed>
- ↑ Roberts, H. L., & Walker, N. (1929). PAUL GERSON UNNA. British Journal of Dermatology, 41(4), 157-160.
- ↑ 41.0 41.1 <pubmed>5556500</pubmed>
- ↑ <pubmed>13206419</pubmed>
- ↑ <pubmed>14441224</pubmed>
- ↑ <pubmed>14003041</pubmed>
- ↑ <pubmed>5267156</pubmed>
- ↑ <pubmed>22549580</pubmed>
- ↑ <pubmed>23147310</pubmed>
- ↑ <pubmed>23739692</pubmed>
- ↑ 49.0 49.1 49.2 49.3 49.4 <pubmed>25284524</pubmed>
- ↑ 50.0 50.1 50.2 50.3 50.4 50.5 50.6 <pubmed>19945622</pubmed>
- ↑ 51.0 51.1 51.2 51.3 <pubmed>24860657</pubmed> Cite error: Invalid
<ref>
tag; name 'PMID24860657' defined multiple times with different content Cite error: Invalid<ref>
tag; name 'PMID24860657' defined multiple times with different content Cite error: Invalid<ref>
tag; name 'PMID24860657' defined multiple times with different content - ↑ <pubmed>19018253</pubmed>
- ↑ <pubmed>23960401</pubmed>
- ↑ 54.0 54.1 54.2 <pubmed>17269961</pubmed>
- ↑ <pubmed>16338213</pubmed>
- ↑ <pubmed>25260052</pubmed>
- ↑ <pubmed>24520234</pubmed>
- ↑ 58.0 58.1 58.2 58.3 58.4 58.5 58.6 <pubmed>19824737</pubmed>
- ↑ 59.0 59.1 59.2 <pubmed>23419760</pubmed>
- ↑ 60.0 60.1 60.2 60.3 <pubmed>24124810</pubmed>
- ↑ <pubmed>21165248 </pubmed>
- ↑ 62.0 62.1 62.2 <pubmed>20682465</pubmed>
- ↑ 63.0 63.1 63.2 63.3 <pubmed>24678015</pubmed>
- ↑ <pubmed>21357618</pubmed>